INTRODUCTION
The inhalation repeated toxicity study evaluates systemic toxicity induced by repeatedly inhaled test substances using experimental animals. During the experimental period, animals are kept in specific chambers equipped for spraying test materials and performing analyses. Control group animals are also kept in chambers supplied with fresh air to maintain the same environmental conditions as the test groups. This specific closed environment could induce stress [1] and the resulting biological changes [2, 3], making it is essential to establish historical data for inhalation toxicity tests.
Clinical pathology including hematology and serum chemistry, reflects pathological or functional changes in various organs [4–6] and is essential for distinguishing between normal and abnormal conditions and evaluating whether it is a toxic change [7, 8]. It is a crucial parameter in several test guidelines for evaluating the toxicity of chemical substances, including test guidelines of the Organization for Economic Cooperation and Development [9–11]. Clinical pathology is also valuable for extrapolating the potential impact on humans.
Historical data provide a basis for understanding the biological characteristics and range of variance in animal species and strains. They help determine whether observed alterations in the toxicity assessment of chemicals, including drugs, are actual or biological changes caused by individual differences [12–15]. Understanding age-related biological characteristics is also essential for interpreting and understanding experimental results.
Recent studies on historical data have focused on neoplastic lesions of 104-week-old rats [16–18]. However, more studies for clinicopathological background data are required to evaluate toxicity for animals in diverse environment. To date, clinicopathological background data for inhalation studies have not been reported, and statistical approaches have been limited in age-related trend analysis. Therefore, this study aimes to obtain background clinicopathological data for F344 rats of different week-ages used in inhalation toxicity studies. In addition, we statistically analyzed age-dependent trends in the data collected to determine aging changes in clinical pathology.
MATERIALS AND METHODS
Six-week old male and female F344/NSlc rats from a specific pathogen-free colony were purchased from Japan SLC (Hamamatsu, Japan) via Joongang Experimental Animal (Seoul, Korea) for use as the control group in 4-, 13-, 26-, and 52-week repeated inhalation toxicity studies. The rats were used after 1 week of quarantine and acclimatization. They rats were housed in a room maintained at 22°C ± 3°C with 50% ± 20% relative humidity, artificial lighting from 08:00 to 20:00 hr, and 12–15 air changes/hr. The rats were housed individually in wire-bottomed stainless-steel mesh cages placed in exposure chambers and provided sterilized tap water and commercial rodent chow (Teklad Certified Irradiated Global 18% Protein Rodent Diet; Envigo, Indianapolis, IN, USA) ad libitum. Rats were exposed to clean dry air for 6 hr/d, 5 d per week for 4, 13, 26, or 52 weeks in whole-body chambers. The study protocols were approved by the Institutional Animal Care and Use Committee (IACUC) of the Inhalation Toxicity Research Center (IACUC approval numbers: IACUC-1911, -1913, -1915, -1916, -1917, -1918, -1812, -1813, -1815, -1817, -1818, -1612, -1601).
All rats were fasted overnight before blood sample collection and anesthesia with isoflurane preceded euthanasia. Euthanasia was peformed by cutting the abdominal aorta and caudal vena cava after blood collection. Blood samples (7–8 mL) were collected from the abdominal aorta. Approximately 3 mL of each blood sample was placed in EDTA-containing vacutainers for hematological measurements. Subsequently, approximately 0.5–1 mL of blood mixed with 3.2% sodium citrate was centrifuged at 3,000 rpm for 10 min at 4°C to measure prothrombin time (PT) and activated partial thromboplastin time (APTT). Blood samples for blood chemistry analysis were also centrifuged at 3,000 rpm for 10 min at 4°C to obtain serum within 1 hr of sample collection.
Measured hematology parameters included erythrocytes s(red blood cell, RBC), hemoglobin (HGB) concentration, hematocrit (HCT), mean cell volume (MCV), mean cell hemoglobin (MCH), mean cell hemoglobin concentration (MCHC), platelet (PLT), leucocytes (white blood cell, WBC), differential WBC count (neutrophils [NEUA], lymphocytes [LYMA], monocytes [MONA], eosinophils [EOSA], and basophils [BASA]), and each cell-to-WBC ratio expressed as neutrophil percentage (NEU%), lymphocyte percentage (LYM%), monocyte percentage (MON%), eosinophil percentage (EOS%), basophil percentage (BAS%), respectively. Reticulocyte count (RETA) and the reticulocyte-to-RBC ratio, expressed as the reticulocyte percentage (RET%), were also measured, along with PT and APTT. Hematological parameters, excluding PT and APTT, were analyzed using ADVIA 2120i (Siemens, Munich, Germany), whereas PT and APTT were analyzed using ACL ELITE systems (Instrumentation Laboratory, Bedford, MA, USA).
The biochemical parameters measured included alanine aminotransferase (ALT), aspartate aminotransferase (AST), alkaline phosphatase (ALP), blood urea nitrogen (BUN), creatinine (CREA), total bilirubin (TBIL), total protein (TP), albumin (ALB), albumin/globulin (A/G) ratio, total cholesterol (TCHO), triglyceride (TG), glucose (GLU), potassium (K), calcium (Ca), chloride (Cl), inorganic phosphorus (IP), and sodium (Na). These biochemical parameters were analyzed using a TBA-120FR automated clinical analyzer (Toshiba, Tokyo, Japan).
Data are presented as means ± S.D. Statistical analyse were performed using the SPSS Statistics version 28 software (IBM, Armonk, NY, USA). Differences between data were statistically evaluated. The homogeneity of variance was determined using Levene’s test. Homogeneous and heterogeneous data were compared using one-way analysis of variance (ANOVA) and non-parametric Kruskal–Wallis test, respectively. If statistical significance (p<0.05) was observed, Dunnett’s test (for ANOVA) or Dunn’s test (for Kruskal–Wallis) was used to compare 4-week data with those of 13-, 26-, and 52-week. In addition, correlation and simple linear regression analyses were performed to determine the relationship between age and each parameter. A simple linear regression analysis was performed on parameters that showed a significant relationship with the experimental week. Regression analysis was performed on the parameters that significant in the correlation analysis.
RESULTS
Statistically significant differences were observed in NEUA, EOSA, LYM%, NEU%, MON%, and PLT at 13, 26, and 52 weeks; HCT and MCHC at 26 weeks; LYMA and BAS% at 26 and 52 weeks; EOS% at 13 and 52 weeks; RBC, MCV, MCH, and WBC at 13 and 26 weeks; MONA and PT at 26 weeks; and RETA, RET%, and BASA at 13 weeks compared those at 4 weeks (Table 1). Their p-values are presented in Table 1. The correlation coefficients between experimental week and MCH (p<0.01), WBC (p<0.05), LYMA (p<0.01), NEUA (p<0.01), EOSA (p<0.05), BASA (p<0.01), LYM% (p<0.01), NEU% (p<0.01), EOS% (p<0.01), BAS% (p<0.01), MON% (p<0.01), and PLT (p<0.01) were significant (Table 2). All parameters analyzed using regression tests, except for WBC count, showed significant F values (MCH, LYMA, NEUA, BASA, LYM%, NEU%, EOS%, BAS%, MON%, and PLT: p<0.01, EOSA: p<0.05; Table 3). All parameters analyzed using regression test, except for MCH and WBC count, showed significant t value (MCH, LYMA, NEUA, BASA, LYM%, NEU%, EOS%, BAS%, MON%, and PLT: p<0.01, EOSA: p<0.05; Table 3). R2 value is presented in Table 3.
RBC, red blood cell; HGB, hemoglobin; HCT, hematocrit; MCV, mean cell volume; MCH, mean cell hemoglobin; MCHC, mean cell hemoglobin concentration; RETA, reticulocyte count; RET%, reticulocyte percentage; WBC, white blood cell; LYMA, lymphocyte count; NEUA, neutrophil count; EOSA, eosinophil count; BASA, basophil count; MONA, monocyte count; LYM%, lymphocyte percentage; NEU%, neutrophil percentage; EOS%, eosinophil percentage; BAS%, basophil percentage; MON%, monocyte percentage; PLT, platelet; APTT, activated partial thromboplastin time; PT, prothrombin time.
RBC, red blood cell; HGB, hemoglobin; HCT, hematocrit; MCV, mean cell volume; MCH, mean cell hemoglobin; MCHC, mean cell hemoglobin concentration; RETA, reticulocyte count; RET%, reticulocyte percentage; WBC, white blood cell; LYMA, lymphocyte count; NEUA, neutrophil count; EOSA, eosinophil count; BASA, basophil count; MONA, monocyte count; LYM%, lymphocyte percentage; NEU%, neutrophil percentage; EOS%, eosinophil percentage; BAS%, basophil percentage; MON%, monocyte percentage; PLT, platelet; APTT, activated partial thromboplastin time; PT, prothrombin time.
Statistically significant differences were observed in MCV, MCH, LYMA, LYM%, NEU%, BAS%, and PLT at 13, 26, and 52 weeks; RBC, RETA, WBC, and BASA at 26 and 52 weeks; EOS% at 52 weeks; MCHC, RET%, PT, and APTT at 26 weeks; and HGB, HCT, and NEUA at 13 weeks compared with those at 4 weeks (Table 4). Their p-values are presented in Table 4. The correlation coefficients between experimental week and RBC count (p<0.01), MCV (p<0.01), MCH (p<0.01), WBC count (p<0.01), LYMA (p<0.01), EOSA (p<0.01), BASA (p<0.01), LYM% (p<0.01), NEU% (p<0.01), EOS% (p<0.01), BAS% (p<0.01), MON% (p<0.05), PLT (p<0.01), APTT (p<0.01), and PT (p<0.01) were significant (Table 2). All parameters analyzed using the regression test showed significant F- (RBC, MCV, MCH, WBC, LYMA, EOSA, BASA, LYM%, NEU%, BAS%, PLT, and APTT: p<0.01, EOS%, MON%, and PT: p<0.05) and t-values (RBC, MCV, MCH, WBC, LYMA, EOSA, BASA, LYM%, NEU%, BAS%, PLT, APTT, and PT: p<0.05, EOS% and MON%: p<0.05; Table 5). R2 value are presented in Table 5.
RBC, red blood cell; HGB, hemoglobin; HCT, hematocrit; MCV, mean cell volume; MCH, mean cell hemoglobin; MCHC, mean cell hemoglobin concentration; RETA, reticulocyte count; RET%, reticulocyte percentage; WBC, white blood cell; LYMA, lymphocyte count; NEUA, neutrophil count; EOSA, eosinophil count; BASA, basophil count; MONA, monocyte count; LYM%, lymphocyte percentage; NEU%, neutrophil percentage; EOS%, eosinophil percentage; BAS%, basophil percentage; MON%, monocyte percentage; PLT, platelet; APTT, activated partial thromboplastin time; PT, prothrombin time.
RBC, red blood cell; MCV, mean cell volume; MCH, mean cell hemoglobin; WBC, white blood cell; LYMA, lymphocyte count; EOSA, eosinophil count; BASA, basophil count; LYM%, lymphocyte percentage; NEU%, neutrophil percentage; EOS%, eosinophil percentage; BAS%, basophil percentage; MON%, monocyte percentage; PLT, platelet; APTT, activated partial thromboplastin time; PT, prothrombin time.
Statistically significant differences were observed in ALP, TP, ALB, A/G ratio, TG, TCHO, and IP at 13, 26, and 52 weeks; ALT, AST, and Cl at 26 and 52 weeks; GLU at 13 and 26 weeks; CREA at 52 weeks; and Na and Ca at 26 weeks compared with those at 4 weeks (Table 6). Their p-values are presented in Table 6. The correlation coefficients between experimental week and ALT (p<0.01), AST (p<0.01), ALP (p<0.01), TP (p<0.01), ALB (p<0.01), A/G ratio (p<0.01), TG (p<0.01), TCHO (p<0.01), GLU (p<0.01), BUN (p<0.05), CREA (p<0.05), Na (p<0.01), Cl (p<0.01), and IP (p<0.01) levels were significant (Table 7). All parameters analyzed using the regression test showed significant F- and t-values (ALT, AST, ALP, TP, ALB, A/G ratio, TG, TCHO, GLU, Na, Cl, and IP: p<0.01, BUN and CREA: p<0.01; Table 8 and Fig. 1). R2 value was presented in Table 8.
ALT, alanine aminotransferase; AST, aspartate aminotransferase; ALP, alkaline phosphatase; TBIL, total bilirubin; TP, total protein; ALB, albumin; A/G, albumin/globulin; TG, triglyceride; TCHO, total cholesterol; GLU, glucose; BUN, blood urea nitrogen; CREA, creatinine; Na, sodium; Cl, chloride; K, potassium; Ca, calcium; IP, inorganic phosphorus.
ALT, alanine aminotransferase; AST, aspartate aminotransferase; ALP, alkaline phosphatase; TBIL, total bilirubin; TP, total protein; ALB, albumin; A/G, albumin/globulin; TG, triglyceride; TCHO, total cholesterol; GLU, glucose; BUN, blood urea nitrogen; CREA, creatinine; Na, sodium; K, potassium; Cl, chloride; Ca, calcium; IP, inorganic phosphorus.
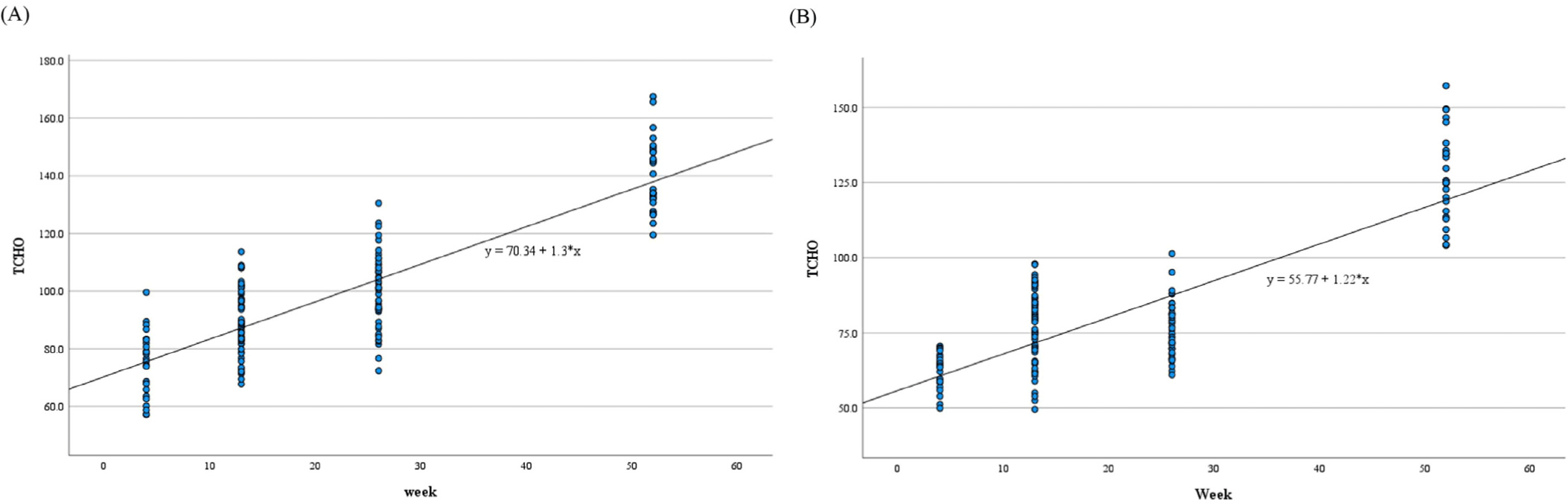
Statistically significant differences were observed in ALP, TP, ALB, A/G ratio, TCHO, and IP at 13, 26, and 52 weeks; ALT, TG, CREA, and Ca at 26 and 52 weeks; AST at 13 and 52 weeks; GLU at 52 weeks; and TBIL, Na, Cl, and K at 26 weeks compared with those at 4 weeks (Table 9). Their p-values are presented in Table 9. The correlation coefficients between experimental week and ALT (p<0.01), AST (p<0.01), ALP (p<0.01), TBIL (p<0.01), TP (p<0.01), ALB (p<0.01), A/G ratio (p<0.01), TG (p<0.01), TCHO (p<0.01), GLU (p<0.01), BUN (p<0.01), CREA (p<0.01), Na (p<0.01), Cl (p<0.01), Ca (p<0.01), and IP (p<0.01) were significant (Table 7). All parameters analyzed using the regression test showed significant F- and t-values (F- and t-values for ALT, AST, ALP, TBIL, TP, ALB, A/G ratio, TG, TCHO, GLU, BUN, CREA, Na, Cl, Ca, and IP: p<0.01; Table 10 and Fig. 1). R2 value are presented in Table 10.
ALT, alanine aminotransferase; AST, aspartate aminotransferase; ALP, alkaline phosphatase; TBIL, total bilirubin; TP, total protein; ALB, albumin; A/G, albumin/globulin; TG, triglyceride; TCHO, total cholesterol; GLU, glucose; BUN, blood urea nitrogen; CREA, creatinine; Na, sodium; Cl, chloride; K, potassium; Ca, calcium; IP, inorganic phosphorus.
ALT, alanine aminotransferase; AST, aspartate aminotransferase; ALP, alkaline phosphatase; TBIL, total bilirubin; TP, total protein; ALB, albumin; A/G, albumin/globulin; TG, triglyceride; TCHO, total cholesterol; GLU, glucose; BUN, blood urea nitrogen; CREA, creatinine; Na, sodium; Cl, chloride; Ca, calcium; IP, inorganic phosphorus.
DISCUSSION
We collected background clinicopathology data for an inhalation study and described trends and alterations in hematological and biochemical parameters with aging in F344 rats.
Statistical significance in correlation analysis with age was noted in 27 parameters in males and 31 parameters in females for hematology and clinical chemistry. Regression analysis was performed on parameters showing the statistical significance in correlation analysis. Positive correlation coefficients indicate an increasing tendency and a direct linear relationship, whereas negative values indicate a decreasing tendency and an inverse linear relationship [19, 20]. Correlation and linear regression analyses revealed a direct linear relationship with age in both sexes for MCH, NEU%, EOS%, MON%, ALT, AST, TP, ALB, TG, TCHO, GLU, BUN, CREA, Na, Cl and an inverse linear relationship for PLT, LYMA, BASA, LYM%, BAS%, ALP, A/G ratio, and IP. Females additionally showed a direct linear relationship with MCV, APTT, and Ca and an inverse linear relationship with RBC, PT, WBC, EOSA, and TBIL with increasing weeks. Males showed a direct linear relationship with NEUA and EOSA, with an increase in weeks. However, most parameters, except for TCHO (R2 = 0.652 in males, R2 = 0.728 in females) and MCV (R2 = 0.503) and TP (R2 = 0.567) in females, had R2 values below 0.5. In addition, these results indicated that although aging is one of the factors affecting values, there are other more affectable factors to the values. Several factors, including aging pathobiology [21, 22], spontaneous changes [23, 24], and sample size, could affect data on clinical pathological parameters.
The intersection of results for mean comparison and regression analysis was examined to confirm change trends with increasing age. Mean comparison results identified parameters that showed significant differences from the 4-week mark across all weeks. LYM%, NEU%, PLT, ALP, TP, ALB, A/G ratio, TCHO, and IP in both sexes, LYMA in females, and NEUA, EOSA, MON%, and TG in males exhibited similar change patterns between mean comparison and regression analyses. NEUA, EOSA, NEU%, MON%, TP, ALB, TG, and TCHO increased, whereas LYMA, LYM%, PLT, ALP, A/G ratio, and IP decreased in both analyses. Parameters with R2 values greater than 0.5 in regression analysis among the common significant parameters in both mean comparison and regression analysis were TCHO in both sexes and TP in females. Therefore, we considered that TCHO is the parameter most affected by aging in both sexes. Age-related changes in TCHO have also been reported in humans, with suggested causes being decreased low-density lipoprotein receptor and the conversion of cholesterol to bile acid with aging [25, 26].
Until now, most studies on trends in historical data have not used statistical analysis or only performed mean comparisons [27–29]. We conducted a mean comparison and regression analysis to clarify the relationships with aging. The results showed that mean difference comparison and trend analysis did not yield exactly the same results; however, they showed similar pattern and could be complementary. Therefore, we propose applying both mean comparison and regression analyses together for trend analysis.
In this study, the clinicopathological background data of F344 rats used in inhalation toxicity studies were presented. To our knowledge, we are the first to present the clinicopathological historical data for inhalation study and statistically analyze the age-related change trends. We confirmed aging trends in clinicopathological parameters and concluded that TCHO is most affected by aging under condition of this study. Further research is required to understand how age affects clinicopathological parameters. These results will be helpful for study design and data interpretation in the field of research using rats.