INTRODUCTION
1,2-Dichlorobenzene (CAS No. 95-50-1, o-dichlorobenzene or o-DCB) is a colorless, halogenated aromatic liquid compound known as a dichlorobenzene isomer, which includes 1,3-dichlorobenzene (meta-dichlorobenzene) and 1,4-dichlorobenzene (para-dichlorobenzene). This compound is primarily used as a base for herbicides, pesticides, solvent in wax, resin, tar, rubber, oil, and paint [1, 2].
This substance is classified as an acute toxic agent 4, eye irritant 2, and skin irritant 2, and has specific target organ toxicity (single) according to the globally harmonized system of classification and labeling of chemicals health hazards categories, and about 10,000–100,000 tonnes per year are manufactured and/or imported in the European Economic Area [3]. The American Conference of Governmental Industrial Hygienists (ACGIH) recommended an exposure limit of 25 ppm as the time-weighted and 50 ppm as the short-term exposure limit. The Occupational Safety and Health Administration (OSHA) and National Institute for Occupational Safety and Health (NIOSH) recommended 50 ppm as the permissible exposure limit-ceiling or recommended exposure limit-ceiling, respectively [4]. 1,2-Dichlorobenzene showed a high level of liver toxicity, causing mortality in an acute oral study and animal inhalation studies using rats and mice [5, 6].
Several researchers also studied differences in liver microsomal metabolism between humans and mice through pharmacokinetic model studies, but further studies are needed because these studies are insufficient to use as evidence for risk assessment [2, 7]. We identified unexpected differences in hepatotoxicity between male and female animals through a subacute inhalation toxicity study, which encouraged us to study the risk of 1,2-dichlorobenzene exposure to occupational workers. While there are some toxicologic data from animal studies on this substance, our study investigated subchronic inhalation toxicity using laboratory mice, which is the main route of exposure to workers, and confirmed differences in specific organ toxicity in male and female mice.
MATERIALS AND METHODS
Six-week-old male and female specific-pathogen-free B6C3F1 mice were purchased (Japan SLC, Shizuoka, Japan) and acclimatized for nine days in polycarbonate cages. We followed the methods of Cho et al. 2020 for the inhalation toxicity study [8]. Exposures were conducted in whole-body inhalation chambers with attached temperature and humidity control devices (Model No. WITC-06M, HCT, Icheon, Korea). All animals were maintained in individual wire mesh cages with three chambers, one for each concentration of the test substance and one control chamber with HEPA-filtered clean air. The ambient temperature and relative humidity of the chamber were 22 ± 3°C and 50 ± 20%, respectively, with a 12:12 hour light/dark cycle with 150~300 lux of illumination. The mice received rodent chow (2918C, Envigo RMS, Indianapolis, IN, USA) and tap water ad libitum. We also provided wooden chew sticks to each mouse as an environmental enrichment item. All animal care and inhalation studies were performed under Good Laboratory Practice (GLP) conditions with modified OECD guidelines (TG413) [9]. The animal study protocol was approved by the Committee on Animal Research Committee of the Occupational Safety and Health Research Institute (Approval No. IACUC-1816).
1,2-Dichlorobenzene (99.0% pure, Lot No.: 010917,012618; CAS No.:95-50-1) was purchased from Samchun Pure Chemicals (Pyeongtaek, Korea). The Environmental conditions were monitored every 30 min (Model No. ITC manager, HCT). We generated the concentration of 1,2-DCB in each chamber using a liquid vapor generator (LVG-04-A, HCT) at least three times during each exposure day and also analyzed the concentration of the test substance using gas chromatography (TRACE1310, Thermo Fisher Scientific, Waltham, MA, USA). The maximum concentration (120 ppm) of 1,2-DCB used in this study was selected based on results from a preliminary subacute inhalation study.
The subchronic inhalation toxicity test was performed following the protocol described by OECD guidelines (TG413), with modifications. A total of 40 mice were randomly assigned to four groups for each sex (n = 10): control (filtered air), T1 (30 ppm), T2 (60 ppm), and T3 (120 ppm). All mice were exposed to 1,2-DCB or filtered air for six hours/day, five days/week for 13 weeks. Body weight data were collected twice a week for six weeks and then once a week until 13 weeks. Individual food consumption were also measured once a week, and clinical observations were recorded twice a day during all exposure periods.
At the end of the study, all animals were anesthetized with isoflurane (I-Fran liquid, Hana Pharm, Seongnam, Korea) and euthanized by exsanguination from the abdominal aorta and vein after blood sampling from the caudal vena cava. The blood samples were collected in EDTA2K-containing sample tubes or serum separation tubes, then analyzed for hematology or serum biochemical tests after centrifugation at 1,800 × g for 10 min to obtain serum. At the necropsy of all animals, organs, including the adrenal glands, brain, epididymides, heart, kidneys, liver, lung, ovaries, spleen, testes, thymus, and uterus, were examined and weighed. The following organs and tissues were also examined, collected, and fixed in 10% neutral buffered formalin or Davison’s fixative (only for the testes and eyes/optic nerves) for histopathologic evaluation: aorta, bone marrow, cecum, colon, duodenum, esophagus, eyes/optic nerves, femur, gallbladder, Harderian glands, ileum, jejunum, larynx, lymph nodes (tracheobronchial and mesenteric), mammary gland, nasopharyngeal tissue, pancreas, parathyroids, pituitary, prostate, rectum, salivary glands (submandibular, sublingual, and parotid), sciatic nerve, seminal vesicle, skeletal muscle, skin, spinal cord, sternum, stifle joint, stomach, teeth, thyroid, tongue, urinary bladder, and vagina. Part of the liver tissue was also resected before fixation and kept at –80°C until relevant examination.
Hematologic and biochemical blood examinations were performed using a hematology analyzer (ADIVA 2120i, Siemens Diagnostics, Tarrytown, NY, USA) or blood chemistry analyzer (TBA-120FR, Toshiba, Tokyo, Japan) for the following parameters: white blood cell counts with differential counts (neutrophils, lymphocytes, monocytes, eosinophils, and basophils), red blood cell count, hemoglobin, hematocrit, mean corpuscular volume, mean corpuscular hemoglobin, mean corpuscular hemoglobin concentration, platelet count, and reticulocytes; and biochemical parameters, including alanine aminotransferase (ALT), aspartate aminotransferase (AST), alkaline phosphatase (ALP), total protein, albumin, globulin, triglycerides (TG), total cholesterol, total bilirubin, blood urea nitrogen, creatinine, sodium, chloride, potassium, calcium, and inorganic phosphorus.
Fixed organs and tissues from the controls and T3 group and the liver and nasal cavities of the T1 and T2 groups were routinely processed for paraffin embedding. Four μm thick tissue was prepared and stained with hematoxylin and eosin (Automatic Stainer, DAKO, Glostrup, Denmark). Five-um thick liver tissue sections were prepared and stained with a trichrome staining kit (Ventana Benchmark Special Stains, Roche, Indianapolis, IN, USA) according to the manufacturer’s instructions. Immunostaining was conducted using EnVisionTM Detection System kit according to the manufacturer’s instructions (Peroxidase/DAB+, Rabbit.Mouse, K5007, DAKO). Briefly, 4-um thick paraffin-embedded liver sections were prepared and deparaffinized, hydrated, and blocked for endogenous peroxidase. Then, heat-induced epitope retrieval was conducted using a pressure cooker with citrate buffer. Cytochrome P450 2E1 and 3A4 antibodies are used for IHC. The microscopic features of the liver were examined and photographed under a light microscope (DM3000, Leica, Wetzlar, Germany). The intensity of immunostaining for CYP2E1 and 3A4 in the central vein area was analyzed in representative images of the serial sections at 20 ×, with five fields for each animal, using the ImageJ program (NIH, Bethesda, MD, USA).
Lipid peroxidation levels were measured in liver homogenates as levels of malondialdehyde (MDA) and 4-hydroxynonenal (4-HNE), the parameters of oxidative stress. Each assay was conducted using kits (OxiSelectTM MDA Adduct Competitive ELISA kit or OxiSelectTM HNE Adduct Competitive ELISA kit, Cell Biolabs, San Diego, CA, USA) following the manufacturer’s instructions.
The data are expressed as the mean ± S.D. Statistical analyses were performed using the Pristima® system (Version 2.0; Xybion, Princeton, NJ, USA) to analyze parameters, including body weight, food consumption, organ weight, and hematological and serum biochemical data. The data were analyzed using Levene’s test for homogeneity of variance and one-way analysis of variance with Dunnett’s multiple test or Kruskal-Wallis with Dunn rank sum test for comparisons between the control and inhaled test substance groups. Statistical analysis of the images and lipid peroxidation were performed using the Student’s t-test (Microsoft Excel, Redmond, WA, USA). A value of p<0.05 or p<0.01 were considered statistically significant.
RESULTS
The actual concentrations of 1,2-DCB in the chambers during the exposure period were measured. The mean (± S.D.) concentrations were 30.04 (± 1.06), 59.95 (± 1.97), and 122.83 (± 13.67) ppm for nominal concentrations of 30, 60, and 120 ppm, confirming the tested concentrations of 1,2-DCB.
During the 90-day study period, there were no animal deaths and adverse clinical signs or decreases in food consumption related with test substance (Data not shown). The mean body weight differences between the control and dosing groups are shown in Table 1. At the end of the study, there were significant differences or tendencies toward decreased body weights in the T1, T2, and T3 groups of male and female mice compared to the control group.
The hematology and blood biochemistry data are shown in Tables 2, 3, 4, and 5. In the blood biochemistry data, there was a significant increase in TG in T3 males and ALP in T1, T2, and T3 males compared to control mice. Other changes in hematology or blood biochemistry value were not considered to be test substance-related.
** Dunnett’s LSD test significant at the 0.01 level.
** N, number of animals examined; WBC, white blood cell count; RBC, red blood cell count; HGB, hemoglobin; HCT, hematocrit; MCV, mean corpuscular volume; MCH, mean corpuscular hemoglobin; MCHC, mean corpuscular hemoglobin concentration; PLT, platelets; NEU%, neutrophil relative; LYM%, lymphocyte relative; MON%, monocyte relative; EOS%, eosinophil relative; BAS%, basophil relative; NEUA, neutrophil absolute; LYMA, lymphocyte absolute; MONA, monocyte absolute; EOSA, eosinophil absolute; BASA, basophil absolute; RET%, reticulocyte relative; RETA, reticulocyte absolute.
N, number of animals examined; WBC, white blood cell count; RBC, red blood cell count; HGB, hemoglobin; HCT, hematocrit; MCV, mean corpuscular volume; MCH, mean corpuscular hemoglobin; MCHC, mean corpuscular hemoglobin concentration; PLT, platelet; NEU%, neutrophil relative; LYM%, lymphocyte relative; MON%, monocyte relative; EOS%, eosinophil relative; BAS%, basophil relative; NEUA, neutrophil absolute; LYMA, lymphocyte absolute; MONA, monocyte absolute; EOSA, eosinophil absolute; BASA, basophil absolute; RET%, reticulocyte relative; RETA, reticulocyte absolute.
N, number of animals examined; Na, sodium; K, potassium; Cl, chloride; TP, total protein; ALB, albumin; CREA, creatinine; BUN, blood urea nitrogen; GLU, glucose; Ca, calcium; IP, inorganic phosphorus; TBIL, total bilirubin; TCHO, total cholesterol; TG, triglycerides; AST, aspartate aminotransferase; ALT, alanine aminotransferase; ALP, alkaline phosphatase; A/G ratio, albumin/globulin ratio.
N, number of animals examined; Na, sodium; K, potassium; Cl, chloride; TP, total protein; ALB, albumin; CREA, creatinine; BUN, blood urea nitrogen; GLU, glucose; Ca, calcium; IP, inorganic phosphorus; TBIL, total bilirubin; TCHO, total cholesterol; TG, triglycerides; AST, aspartate aminotransferase; ALT, alanine aminotransferase; ALP, alkaline phosphatase; A/G ratio, albumin/globulin ratio.
The absolute and relative (% for terminal body weight) organ weights are shown in Tables 6 and 7. There were some differences in organ weights between the controls and dosing groups, but these were not considered test substance-related adverse changes.
Macroscopic findings, including liver spots, were observed in male T3 mice (Table 8), which were correlated with mineralization on histopathological findings. The microscopic findings in the liver and nasal cavities of male and female mice are summarized in Table 9. Dystrophic mineralized deposits, necrosis, and pigmentation of the liver were observed in male T3 mice, and Masson’s trichrome stain revealed a fibrotic region surrounding the mineralization and necrosis (Fig. 1). Eosinophilic globules, basal cell hyperplasia, and respiratory metaplasia of the nasal cavity were also observed in 1,2-DCB-inhaled male and female mice (Table 9). Other macro and microscopic findings were within the range of normal background alterations seen in animals of this strain and age and were not related to the test substance.
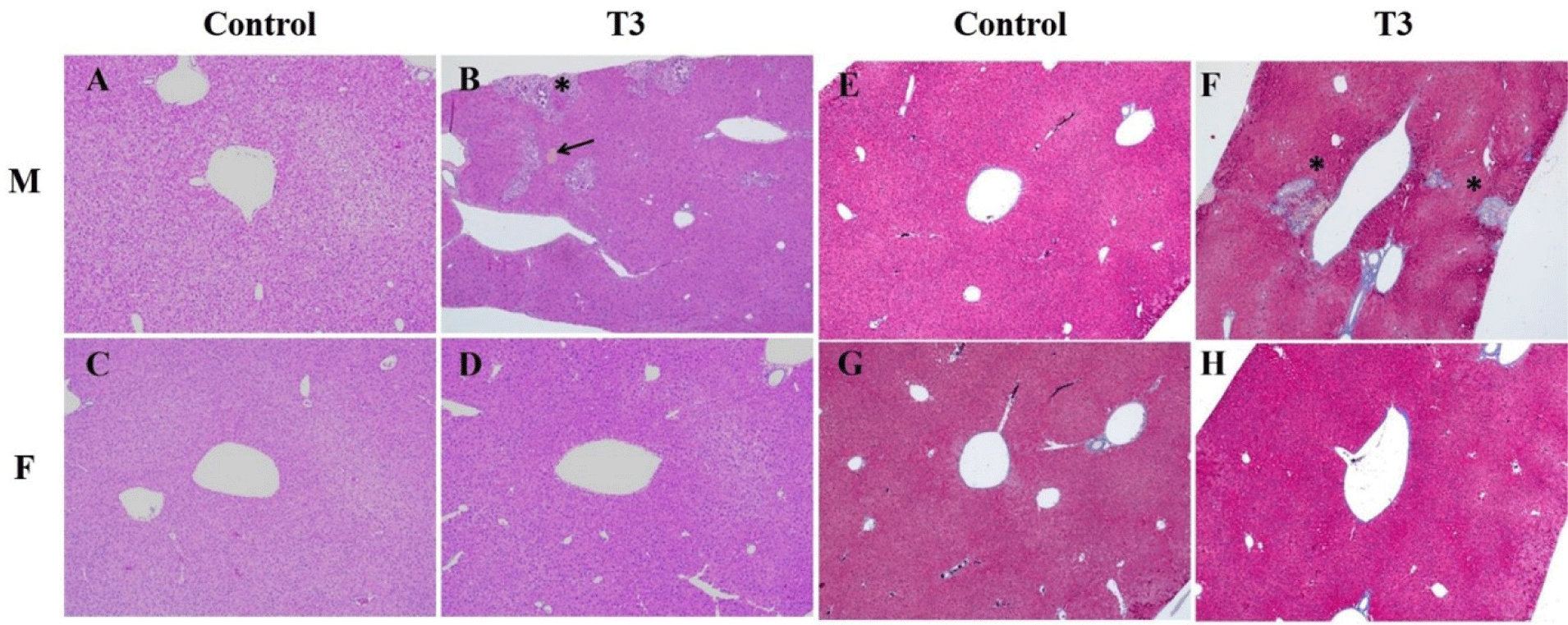
The immunohistochemical analysis of CYP protein expression is shown in Fig. 2. An obvious expression of CYP2E1 and CYP3A4 was detected in the centrilobular area of the livers of male and female mice in the T3 group compared to the control group (p<0.05). In addition, CYP450 expression was increased in male T3 mice compared to female T3 mice (p<0.05).
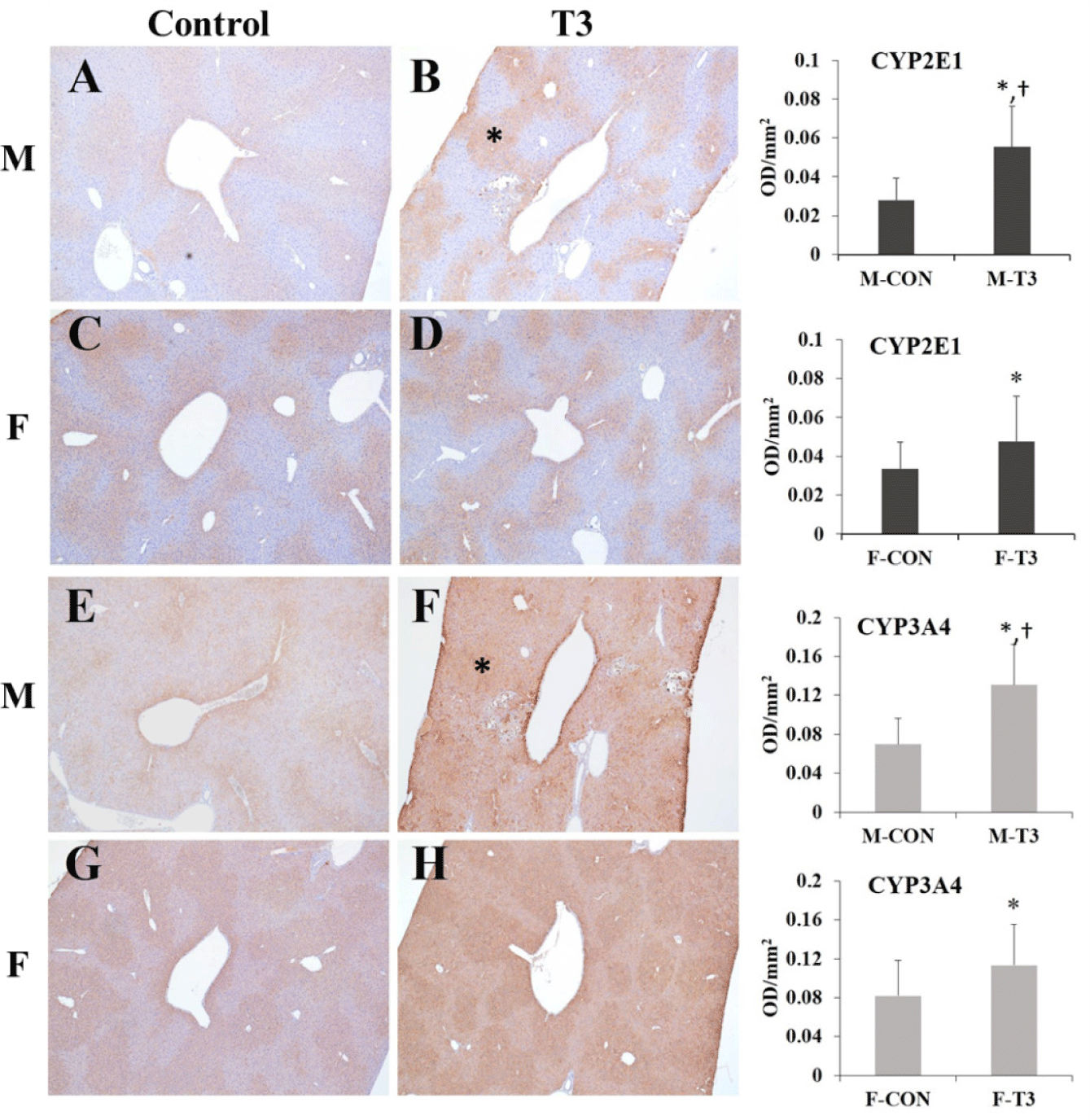
MDA and 4-HNE levels, indicating lipid peroxidation products, were analyzed in liver tissue (Fig. 3). A slightly increasing trend was observed in MDA in the T3 group of male and female mice, and an increased trend in 4-HNE was observed in male T3 group mice, although not statistically significant.
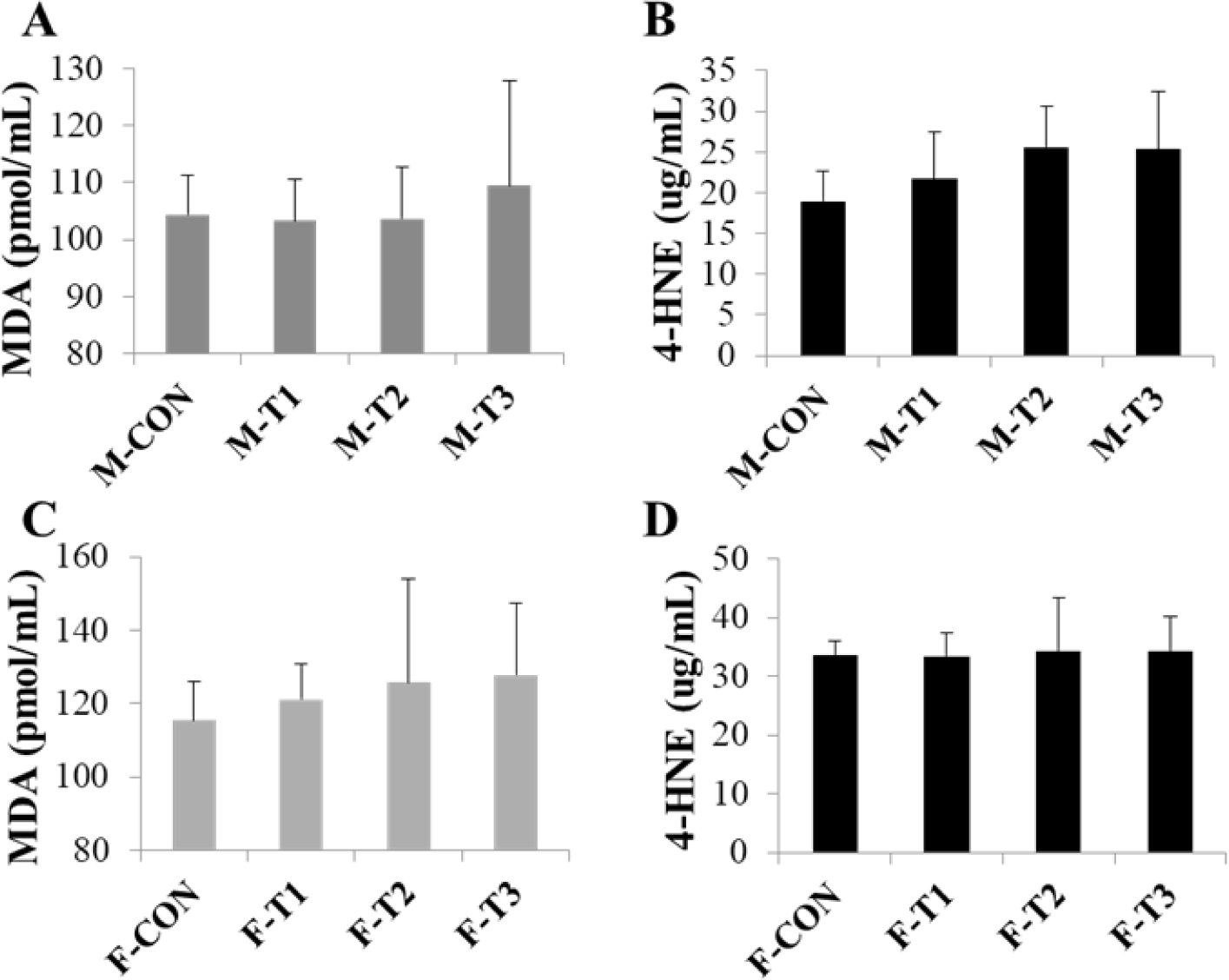
DISCUSSION
There are few restricted data concerning the effects of 1,2-DCB or mixture containing 1,2-DCB such as irritation to the eyes and respiratory passages, hematopoietic disorder, headache, nausea and malaise in workers [3, 10]. Data on health hazards of 1,2-DCB were mostly identified as results of animal or in vitro experiments and there were no reports of on volunteers [10]. In the toxicity of 1,2-DCB, a subchronic oral study using Sprague-Dawley rats reported histopathologic lesions in the liver, such as centrilobular degeneration and centrilobular hypertrophy in both sexes at high doses, single-cell necrosis in males, and necrosis in females. In the clinical biochemistry results, an increase in ALT in middle-dose rats was not accepted because there was no histopathologic evidence, dose-dependency, or change in other enzymes that indicated liver damage [11]. Similar to the results of the above study, in another oral study using F344 rats, hepatotoxicity was identified, including centrilobular degeneration, single-cell necrosis, and necrosis of the liver in high-dose rats of both sexes. Body weight decreases in high-dose males, and relative liver weight increases in middle and high-dose males and females were also noted for determination of no-observed-adverse-effect-level (NOAEL) [6, 12]. Chronic and carcinogenic toxicity studies of 1,2-DCB oral exposure in F344 rats reported the occurrence of tumors, including adrenal gland pheochromocytomas and interstitial-cell tumors of the testis in males, but these have not been recognized as carcinogenic test substances [6, 12]. In mouse oral toxicity studies, the high-dose administration of 1,2-DCB induced body weight decreases, liver lesions, such as centrilobular necrosis, single-cell necrosis, hepatocellular degeneration, mineral deposits in the heart, and lymphoid depletion in the thymus and spleen in both sexes. Pigmentation, hepatocellular degeneration, and single-cell necrosis of the liver were observed in middle-dose males [6, 12]. Chronic and carcinogenic studies revealed non-neoplastic kidney lesions considered to be test substance-related in male mice and malignant histiocytic lymphomas and alveolar/bronchiolar carcinomas, which were considered not to be related to the test substance [6, 12]. A few reports using the inhalation test route in animals, including rats, guinea pigs, and rabbits, reported body weight loss, spleen weight decreases, and lesions in the liver and kidneys. An inhalation study using Swiss male mice exposed to 1,2-DCB (64 and 163 ppm) reported the induction of upper respiratory damage, including damaged olfactory epithelium, which can regenerate [13].
In our preliminary study conducted to determine test concentrations, dosing with 150 ppm led to the death of the male mice (2 of 5) or liver lesions, including hepatic necrosis, accompanied by increases in biochemical enzymes, such as AST and ALT, in the surviving mice. Since liver toxicity was not confirmed in females, differences in enzymatic expression and lipid peroxidation in the liver between the sexes were confirmed in an additional 90-day inhalation study. In the subchronic study, exposure to 1,2-DCB (30, 60, 120 ppm) induced slight decreases in the body weight of the mice and liver damage only in the male T3 group without serum biochemical changes or damage of the upper respiratory tract, especially nasal cavity in both sexes of all dosing mice. In addition, fibrous tissue proliferated around the areas of mineralization, and some necrotic areas were identified in the livers.
Liver injury and regeneration induced by chemicals have been well-recognized through many studies. In the short term, the liver can recover normal structure by proliferating or reconstructing the remaining cells. However, prolonged damage causes liver fibrosis, which can cause cirrhosis or liver cancer [14]. In the results of our study using B6C3F1 mice, 1,2-DCB is believed to have caused repeated tissue damage, and individual animals adapted to the initial toxicity after primary metabolism occurred in the liver and recovered. Previous studies showed that the test substance was quickly absorbed and oxidized by CYP450 in the early stages of metabolism to form an intermediate epoxide, which is known to be associated with hepatotoxicity [15]. Some researchers also studied how alterations in CYP450 or glutathione affected hepatotoxicity in animals [16]. Moreover, in the literature on sex differences in the metabolism of 1,2-DCB in rats and mice, the liver microsomes of female rats metabolized 1,2-DCB faster than males, but in contrast, this process was more efficient in male mice than in females. Additionally, the metabolism of the test substance is known to be faster in mice than in rats [17]. CYP450s, key enzymes in the liver metabolism of drugs and chemicals, can produce reactive oxygen species, causing oxidative damage, lipid peroxidation, protein modification, and mitochondrial damage [18–20]. The IHC analysis showed that CYP2E1 and CYP3A4 were expressed around the central vein of the liver in T3 mice, and both males and females showed a significant increase in expression compared to the control group. In addition, the expression of CYPs was significantly increased in males compared to females. In this regard, the analysis of MDA and 4-HNE in the liver confirmed increased changes in male and/or female T3 mice and sex differences.
An evaluation of CYP450 enzymatic activity is important for identifying inhibition or induction characteristics in drug and chemical metabolism, and major isoforms, including 1A2, 2E1, 2C9, 2C19, and 3A have been identified in previous studies [21, 22]. Although there is a difference between humans and animal species, the similarities between human and mouse hepatic-metabolizing characteristics were shown in recent studies and could strengthen our research findings [23]. Thus, despite the need for further studies on the relevance of 1,2-DCB to human, the mechanistic approaches to the toxicity identified in vivo would be useful because health hazards data on human associated with 1,2-DCB could not exclude the effects of accidental exposure or mixture form.
In summary, we conducted 90-day inhalation toxicity studies using male and female B6C3F1 mice to evaluate the toxicity of 1,2-DCB under inhalation exposure conditions in accordance with GLP and OECD guidelines. The present study showed that inhaled 1,2-DCB induced upper respiratory damage in both sexes of mice and hepatotoxicity only in males and that differences in liver toxicity were associated with lipid peroxidation due to CYP450 alterations.