INTRODUCTION
Canine parvovirus (CPV) is a highly contagious virus that spreads among dogs via oronasal exposure or the fecal oral route and causes severe gastrointestinal disease and, occasionally, cardiac disease [1, 2]. Despite the availability and widespread use of effective vaccines, CPV remains a major cause of morbidity and mortality in dogs [3] and no definite treatment for infected animals has been established. At present, treatment of parvoviral enteritis is primarily supportive and focused on ameliorating symptoms. Even with aggressive supportive care, the mortality rate ranges from 4% to 48% [1]. Novel adjunctive drugs have been investigated, but study results have been disappointing or inconsistent [4].
Because CPV exhibits tropism for rapidly replicating cells, cell populations of the intestinal crypt epithelium and lymphoid and hematopoietic tissues can be affected [5]. Infected dogs develop intestinal lesions due to the destruction of enterocytes in the intestinal crypts, accompanied with villus blunting and atrophy [5, 6]. Gut-associated and systemic lymphoid tissues are also affected [5]. Consequently, CPV enteritis may cause destruction of the gut barrier, potentially leading to bacterial and endotoxin translocation with associated bacteremia and endotoxemia. This can lead to the development of a systemic inflammatory response and multiple organ dysfunction syndromes [3, 4].
L-analyl-L-glutamine (Ala-Gln) is a Gln derivative that is more stable than Gln and provides more Gln molecules at the physiological osmolality required for oral solutions [7, 8]. In a previous study, sedentary rats orally administered Ala-Gln exhibited a greater increase in the plasma Gln concentration than rats supplemented with free Gln, and chronic supplementation with Ala-Gln led to larger muscle and liver Gln stores [9]. Ala-Gln is absorbed in its intact form by the luminal membrane via an intestinal transport mechanism for dipeptides that differs from the transport mechanism for free amino acids [10].
Gln is the preferred fuel source for enterocytes [11]. While Gln traditionally has been considered a nonessential amino acid, it is currently regarded as a conditionally essential amino acid in conditions of serious illness, injury, or other catabolic states in which Gln in the plasma and tissues can become depleted [12]. Gln has beneficial effects on the intestinal barrier and modulates the gut-associated immune system [13, 14]. Dietary Gln supplementation decreases the susceptibility of enterocytes and lymphatic cells to apoptosis, and enhances anti-oxidative function and cell proliferation in the swine small intestine [14, 15]. For these reasons, interest in the therapeutic role of Gln has increased in veterinary medicine, and its pharmacological effects have been investigated in both humans and experimental animal models [12, 16]. However, to our knowledge, little is known about the effect of Ala-Gln supplementation in dogs with parvoviral enteritis. The objective of this study was to determine whether Ala-Gln supplementation in dogs with parvoviral enteritis can improve their survival and ameliorate their clinical signs without side effects.
MATERIALS AND METHODS
Client-owned dogs with parvoviral enteritis were recruited between September 2009 and May 2010. The inclusion criteria for enrollment were a positive CPV fecal antigen test (SNAP Parvo antigen test, IDEXX Laboratories, Westbrook, ME, USA), the presence of relevant clinical signs (vomiting, depression, diarrhea, and anorexia), and no treatment prior to enrollment. Exclusion criteria were the presence of fecal oocytes of enteric parasites on fecal flotation, severe anemia (packed cell volume < 20%) and hypoalbuminemia (serum albumin concentration < 2.0 g/dL), or the presence of a concurrent disease. The diagnosis of parvoviral enteritis was confirmed by PCR. Informed consent was received from all dog owners. The dogs were assigned to two study groups in a random and double-blind manner. The study protocol was approved by the ethics committee of Chungbuk National University.
A treatment protocol was designed to standardize therapy for the two groups, with the exception of Ala-Gln supplementation in the treatment group. All patients received supportive care consisting of fluid therapy with potassium chloride supplementation based on laboratory results. The dogs also receive antibiotics, including ampicillin-sulbactam (Sulbacin inj.; Dongkwang Pharmaceutical, Seoul, Korea) and metronidazole (Metrynal inj.; Daihan Pharm, Seoul, Korea), antiemetics (ondansetron [Otron] inj.; Myungmoon Pharm, Seoul, Korea), H2 blocker (famotidine [Gaster] inj.; Dong-A ST, Seoul, Korea), anticoagulant (dalteparin [Fragmin] inj.; Pfizer Manufacturing Belgium N.V./S.A, Puurs, Belgium) and analgesics (butorphanol [Butophan] inj.; Myungmoon Pharm). When an animal’s packed cell volume dropped < 20% or its serum albumin concentration decreased < 2.0 g/dL during hospitalization, the attending clinician administered whole blood or human albumin (human serum albumin 20%, 100 mL, Green Cross, Yongin, Korea) if deemed necessary.
Dogs in the Ala-Gln group (n = 20) received a 20% Ala-Gln solution (0.4 g/kg; Dipeptiven Inj.; Fresenius Kabi Korea, Seoul, Korea) orally twice daily. An equivalent volume of a placebo (0.9% normal saline) was administered to the dogs in the placebo group (n = 19).
A previously published clinical scoring system [4] was used to evaluate four clinical signs in each patient: general attitude, appetite, vomiting, and the appearance of the feces (Table 1). The total clinical score was defined as the sum of the scores for the four signs.
Data were analyzed using GraphPad Prism 5.04 (GraphPad Software, La Jolla, CA, USA). Categorical variables were compared between the two groups using Fisher’s exact test. Differences in continuous variables were assessed using the t-test or Mann‒Whitney rank sum test. Differences at various time points within each group were evaluated using repeated-measures analysis of variance. Survival data were compared using a log-rank test applied to Kaplan‒Meier survival curves. p<0.05 was considered statistically significant.
RESULTS
Thirty-nine dogs were enrolled (n = 20 in the Ala-Gln group and n = 19 in the placebo group; Table 2). Seventeen dogs (n = 8 in the Ala-Gln-treated group and n = 9 in the placebo group) were vaccinated. Demographic data at baseline (age, weight, breed, sex) did not differ between the two groups (Table 2). The study population consisted of 29 purebred and 10 mixed-breed dogs. All dogs received standard treatment while hospitalized and were monitored for clinical signs and evaluated diagnostically daily for 11 days.
At admission, there were no significant differences between the two groups in attitude, appetite, vomiting frequency, fecal appearance, or total clinical score (Fig. 1). The clinical scores for attitude ranged from 1 to 3 (median = 2) in both groups. For appetite, the scores ranged from 0 to 2 in the Ala-Gln group and from 1 to 2 in the placebo group. For vomiting, the scores ranged from 1 to 3 (median = 2 for both groups), and for feces appearance, from 1 to 3 (median = 2 for both groups). Total clinical scores ranged from 3 to 10 in the Ala-Gln group and from 4 to 10 in the placebo group (median = 8 for both groups).
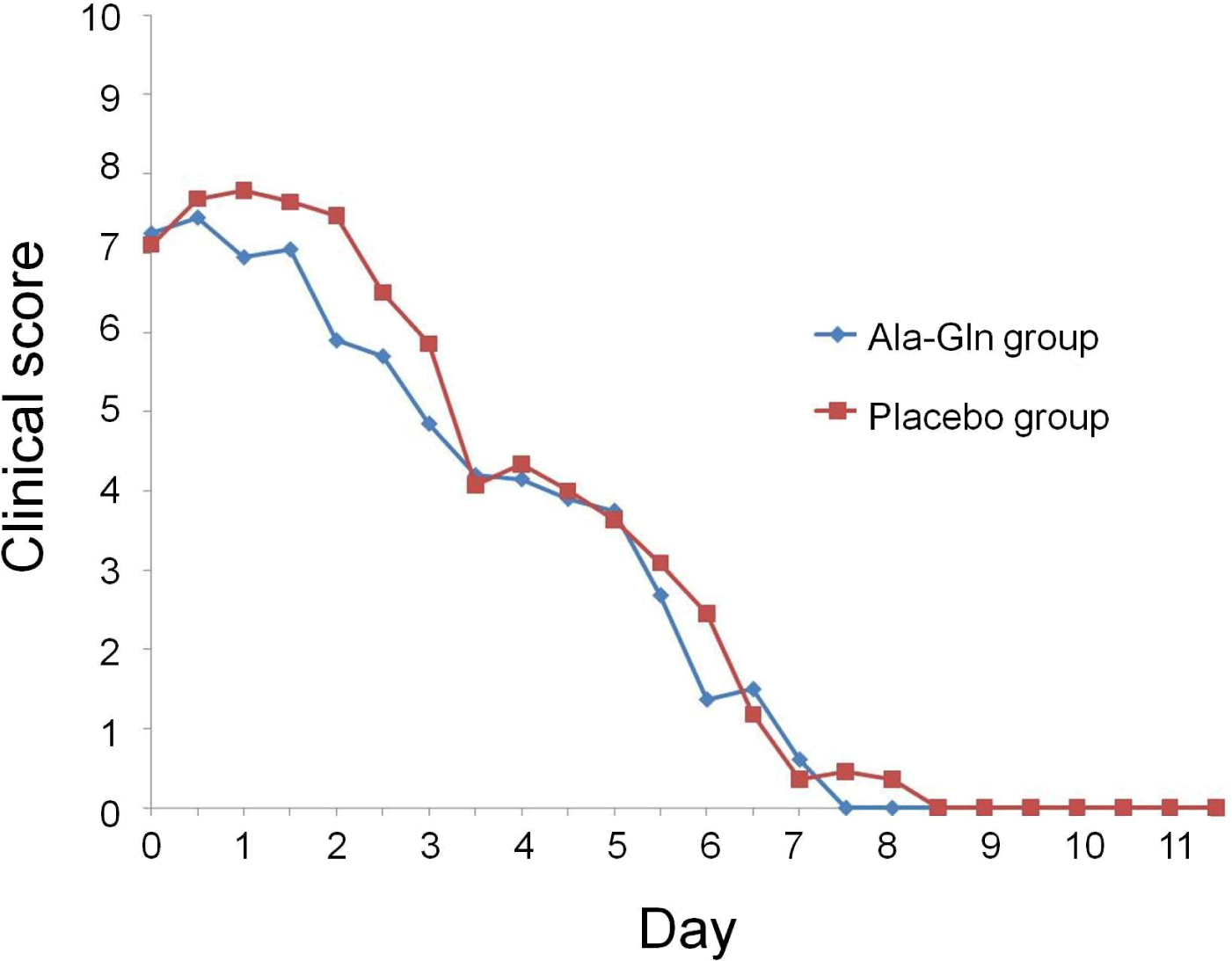
Appetite improved faster in the Ala-Gln group (by day 6) than in the placebo group (p=0.037). There were no differences in the other categorical variables between the two groups. However, the median time required to attain a zero score for all categorical variables (attitude, appetite, vomiting, and diarrhea) was consistently 1 day shorter in the Ala-Gln group than in the placebo group. Compared to those in the placebo group, the clinical scores in the Ala-Gln group were significantly improved by day 2 (p=0.014); however, after day 2, there were no differences.
There was a significant difference in the distribution of survival times between the two groups (p=0.038). Three of the 20 dogs (15%) in the Ala-Gln group died, whereas eight of the 19 dogs (42%) in the placebo group died (Fig. 2). There were no adverse drug reactions associated with the administration of Ala-Gln such as blood dyscrasias, hepatotoxicity, and cutaneous signs.
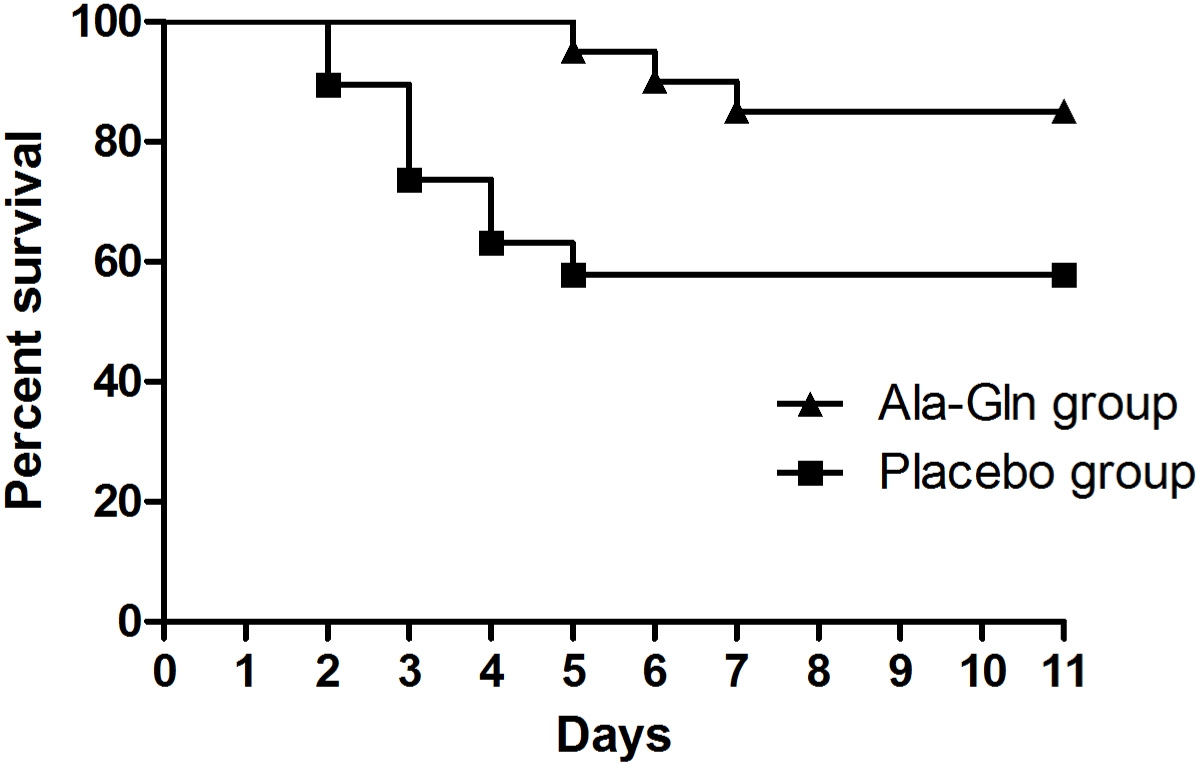
DISCUSSION
The dogs administered Ala-Gln exhibited significantly higher survival than the dogs in the placebo group. However, the mean total score for clinical sign did not differ between the groups, except on day 2. Dogs who died were excluded from the clinical score calculation. In addition, the non-surviving dogs had total clinical scores ≥ 11 before death, suggesting they had severe clinical signs. Therefore, the exclusion of dogs who died may have influenced the mean total clinical score. There was a significant difference in the mean total clinical score in the Ala-Gln group on day 2, implying that the Ala-Gln administration has an early therapeutic effect in therms of clinical improvement. Given that, the survival rate was higher in the Ala-Gln group and there were no adverse effects after the administration of Ala-Gln, Ala-Gln has high potential therapeutic value for the treatment of parvoviral enteritis in dogs.
As the preferred fuel of enterocytes, Gln may reduce bacterial translocation across the gut barrier and therefore, reduce the risk of sepsis [17‒19]. Besides its role as a major fuel for enterocytes, Gln has a number of regulatory effects. Gln has a trophic effect on enterocytes, increasing protein and nucleotide synthesis and reinforcing the mitogenic action of growth factors such as epidermal growth factor and growth hormone. It can also inhibit apoptosis [20‒23]. In addition to these protective and regulatory effects on enterocytes, Gln can be considered an immunoenhancing nutrient. It plays an important role in immune cell mechanism, increasing the function of lymphocytes and macrophages by providing them with a respiratory substrate and nucleotide precursor [24, 25]. The Gln dipeptide Ala-Gln, when supplemented in total parenteral nutrition (TPN), improved survival rates in a rat protracted peritonitis model; rats in the Ala-Gln-supplemented TPN group exhibited 84% survival, whereas rats receiving TPN with a conventional amino acid solution exhibited 40% survival [26]. In line herewith, in the present study, the Ala-Gln group showed a significantly higher survival rate than the placebo group. Our results point to a potential novel adjunctive therapy for dogs with parvoviral enteritis.
Treatment of dogs with parvoviral enteritis is often successful; nevertheless, death may occur because of complications such as sepsis, systemic inflammatory response syndrome, endotoxemia, or disseminated intravascular coagulation [27]. Inflammatory cytokines such as tumor-necrosis factor alpha (TNF-α) play an integral role in parvoviral enteritis and endotoxemia. TNF-α is produced primarily by mononuclear phagocytes and may prime neutrophils to respond to specific activating factors that enhance respiratory bursts, release reactive oxygen species, and trigger phagocytosis [28]. This cytokine and endotoxin may be more important than bacteremia in the progression of clinical signs [29]. In one study, dogs treated with TNF-α developed bloody or watery diarrhea before death [30]. Another study suggested that bloody diarrhea during parvoviral infection results from endotoxemia and cytokine production, not from viral infection [31]. Increased TNF-α levels may be predictive of mortality. Endotoxin activity tended to increase prior to death in dogs with naturally occurring parvoviral enteritis [29]. The results from these previous studies [29, 30] show that endotoxins and cytokine cascade activation are integral to the pathogenesis of canine parvoviral enteritis. Experiments in vivo and in vitro have shown that Gln regulates the immune response and attenuates the release of inflammatory cytokines such as TNF-α in response to oxidative stress, thus preventing lung injury during acute respiratory distress syndrome [32].
After cell entry, Gln is broken down into glutamate and ammonia. Glutamate is an important substrate for glutathione synthesis. Gln may therefore increase glutathione levels and improve the immune function of the gastrointestinal tract [33]. Gln may also prevent the activation of transcription factors such as nuclear factor kappa B in intestinal epithelial cells during sepsis [34]. In humans, Gln reduces pro-inflammatory cytokine production in the intestinal mucosa, probably via a post-transcriptional pathway [32]. Similar results have been observed in rats with experimental colitis [35]. Therefore, Gln may be useful as a therapeutic intervention to modulate imbalanced cytokine production. Intervention with Gln to block endotoxemia and the cytokine cascade may decrease mortality in dogs with parvoviral enteritis. Indeed, it has been reported that parenteral Ala-Gln administration in dogs resulted in an increase in the phagocytic responses of polymorphonuclear neutrophilic leukocytes that were suppressed by high-dose methylprednisolone sodium succinate treatment [36], indicating that Ala-Gln can be used for the treatment of CPV infection.
Interest in the therapeutic role of Ala-Gln has grown recently. In human medicine, research on Ala-Gln has been concentrated in the field of clinical nutrition [37]. As the therapeutic effects of Ala-Gln have not been extensively studied in veterinary medicine, our results will be helpful in designing treatment regimens for various diseases, including canine parvoviral enteritis. In our study, the dose of Ala-Gln (0.4 g/kg) was calculated using an amino acid requirement formula (g/d): 2.3 × body weight in kilograms [36]. This is relevant because an increase in protein intake may be necessary for patients with higher than usual protein requirements and because the metabolic demand for Gln can increase in critically ill patients [12, 38‒40]. In studies on the intestinal metabolism of dietary Gln, the dipeptide Ala-Gln has been used as an alternative form of Gln for oral administration [37]. Ala-Gln is more stable than Gln at low pH and under the high temperatures used to sterilize enteral and parenteral solutions [41‒43]. Furthermore, Ala-Gln solutions provide more Gln molecules at the physiological osmolality inquired for oral rehydration solutions [43]. A previous study indicated that both Ala-Gln and free forms of L-Gln and L-alanine administered after resistance exercise induced cytoprotective effects that were mediated by HSP70-associated responses to muscle damage and inflammation [37]. Ala-Gln-supplemented oral rehydration and nutrition therapy broke the vicious cycle of diarrhea and malnutrition induced by the cholera toxin in a rat model [43]. Therefore, L-alanine supplementation may be important for Gln utilization and metabolism. However, in the present study, serum Gln concentrations were not measured and therefore, the therapeutic dose of Ala-Gln in dogs with parvoviral enteritis could not be estimated. Further study of the role of inflammatory cytokines and immune function in CPV-infected dogs should help clarify the therapeutic effects of Ala-Gln.
This study had several limitations. First, the clinical scoring of attitude and appetite was somewhat subjective. Nevertheless, this scoring system proved to be practical and useful for assessing disease severity in dogs with parvoviral enteritis in a previous study [4]. Moreover, survival rate was clearly higher in the Ala-Gln group, affirming the clinical score results. Second, we did not assess whether a different route of Ala-Gln administration would influence the clinical response. Especially, Dogs in the Ala-Gln group received intravenous solution of Ala-Gln orally. According to a previous study [43], Ala-Gln molecules are transported across the intestinal epithelium by the oligopeptide transporter PepT-1, which exhibits a higher absorption rate for Ala-Gln than for free amino acids. The high intracytoplasmic dipeptidase concentration in enterocytes may favor an increased stoichiometric release of Gln and Ala and thus produce a greater kinetic response to the plasma Gln concentration [43]. Numerous studies on parenteral Gln supplementation have been performed; however, it remains unclear whether the route of Gln administration can determine its beneficial effects [44]. As L-alanine supplementation may be important for Gln metabolism, we cannot conclude that the effect of Ala-Gln supplementation was solely due to Gln [37]. Future studies should focus on determining the therapeutic dose and route of Ala-Gln in dogs with parvoviral enteritis. Finally, we did not focus on the potential side effect of Ala-Gln whether there were no blood dyscrasias, hepatotoxicity, and cutaneous signs which are frequently observed as adverse drug reactions in dogs [45]. Furthermore, it could be not elucidated whether some vomiting, salivation and sedation were due to Ala-Gln or parvoviral enteritis. However, severe adverse drug reactions such as blood dyscrasias, hepatotoxicity, and cutaneous signs were not observed during study period (11 days) and the survival rate of Ala-Gln group were higher than those of placebo group, indicating there might be no clinically relevant adverse drug reactions of Ala-Gln in dogs with parvoviral enteritis although some side effects could be existed.
In conclusion, our results suggest that oral administration of Ala-Gln is effective in improveing the survival of dogs with parvoviral enteritis. However, further population-based studies are required to clarify the effects of Gln in dogs with parvoviral enteritis.