INTRODUCTION
Unhealthy diets and inactive lifestyles have led to a global epidemic of obesity. Over the past few decades, obesity has become a public health problem because of its direct link to the increase in many other health problems, including diabetes, cardiovascular disease, cancer, and infertility. Obesity has also been associated with an increased risk of both all-cause and cause-specific mortality [1]. Previous studies have shown that a high-fat diet (HFD) is responsible for increased adiposity [1, 2]. Fat cells in obese men can produce estrone via aromatization, which inhibits the secretion of gonadotropin, causing endocrine abnormalities and infertility [3]. In addition, diet-induced obesity increases the potential for the accumulation of fat deposits in the suprapubic and scrotal areas [3, 4]. Therefore, it can increase the temperature of the testis and affect sperm production [3–5]. In addition, previous studies have also demonstrated that obesity increases the DNA fragmentation rate in sperm, resulting in azoospermia and oligozoospermia [6, 7].
Silent information regulator 2 (Sir2) proteins, or sirtuins (SIRT) belong to an evolutionarily conserved family of nicotinamide adenine dinucleotide-dependent protein deacetylases. SIRT proteins play an essential role in the post-translational regulation of many metabolic and cyto-protective processes [8]. The mammalian SIRT1 gene belongs to a class III histone deacetylase. It is a mitochondrial protein significantly associated with metabolic control, health span, and longevity [9, 10]. SIRT1 is an essential regulator of energy through its impact on glucose and lipid metabolism [11, 12]. Previous studies have reported that SIRT knockout (KO) mice exhibit decreased energy expenditure and hypersensitivity to HFD [13, 14]. Dietary habits and environmental influences such as exposure to toxins, dioxins, combustion products and radiation have significant consequences on the efficiency of spermatogenesis and a considerable impact on male fertility [15, 16]. Fertility disorders could result from the interruption of all these factors. Therefore, the present study was conducted to examine the alteration of the testicular function of SIRT1 mice on HFD.
MATERIALS AND METHODS
SIRT1 wild type (WT; SIRT1loxP/loxPLysM-Cre–/–) and KO (SIRT1; SIRT1loxP/loxPLysM-Cre+/+) mice were acquired as previously described [17]. Six-weeks-old WT and SIRT1 male mice were fed ad libitum with a control normal chow diet (NC) or a HFD that included 40% kcal from soybean and coconut oil (Research Diets, New Brunswick, NJ, USA) for 32 weeks. The animals were housed in a facility at 22℃–25℃ with a humidity of 50 ± 10% and a 12 hr light/dark cycle. All mouse experiments were performed according to the Institutional Animal Care and Use Committee (IACUC) protocol guidelines and approved by the IACUC of Chungbuk National University, Korea (CBNUR-1626-21).
Unless otherwise noted, all other reagents used in this study were purchased from Sigma-Aldrich Chemical (St. Louis, MO, USA).
Carbon dioxide (CO2) inhalation was used to sacrifice the treated mice in all the groups. Blood was aspirated by a 1 mL syringe, and centrifuged at 500 × g for 10 min. Thereafter, serum was collected and stored at –20℃ for further examination. The liver, testes, and seminal vesicles were excised and immediately weighed on an electronic scale. The dissected epididymis was washed thrice with phosphate-buffered saline (PBS). Afterward, it was minced in 2 mL PBS supplemented with 0.1% polyvinyl alcohol (PBS-PVA) by a surgical blade and placed at 37.5℃ for 10 min. Then, the motility of released sperm was estimated under a light microscope at 400× magnification. A hemocytometer (Marienfeld-Superior, Lauda-Königshofen, Germany) was used to estimate sperm concentrations.
Glutamate oxaloacetate transaminase (GOT) and glutamate pyruvate transaminase (GPT) were assayed by the Reitman-Frankel method [18]. The serum levels of the enzymes were determined using commercial detection kits based on the manufacturer’s instructions (Asan Pharmaceutical, Seoul, Korea).
The serum concentrations of interleukin (IL)-1β (BioLegend, San Diego, CA, USA), IL-6 (Thermo Fisher Scientific, Waltham, MA, USA), and tumor necrosis factor (TNF)-α (Thermo Fisher Scientific) were measured using the respective kits according to the manufacturer’s protocol. The concentrations were inferred using corresponding standards by measuring the optical density value at 450 nm (Absorbance 96, Byonoy GmbH, Hamburg, Germany).
The testosterone competitive enzyme immunoassay (EIA) kit was used to determine the serum testosterone concentrations, according to the protocol suggested by the manufacturer (Cayman Chemical, Ann Arbor, MI, USA). Briefly, the EIA kit was rinsed thrice with washing buffer, and 50 μL testosterone standard and sample were added per well. Testosterone acetylcholinesterase tracer and testosterone antiserum (50 μL each) were incubated on a plate shaker for 2 hrs at room temperature (RT). The wells were emptied and rinsed five times with washing buffer. Ellman’s reagent (200 μL) was added to each well, the plate was incubated for 1 hr, and subsequently read at 405 nm on a microplate reader (Byonoy GmbH).
The total RNA was isolated from the testis tissue using RNAiso Plus (Takara Bio, Shiga, Japan). After homogenization of the tissue samples, the homogenate was extracted with chloroform and then RNA was precipitated with isopropanol. The RNA concentration was measured using a nanodrop spectrophotometer (DeNovix DS-11FX, DeNovix, Wilmington, DE, USA). Complementary DNA (cDNA) was synthesized from purified RNA using the TOYOBO ReverTra Ace qPCR RT kit (TOYOBO, Osaka, Japan) according to the manufacturer’s protocol. The relative gene expressions were measured by a quantitative real-time PCR (MyGo Pro PCR cycler, Diagnostic Technology, Belrose, Australia) with SYBR™ Premix Ex Taq™ II (Bioneer, Daejeon, Korea). The relative expression levels of mRNA from the target genes were compared with that of the endogenous control β-actin. The sequences of the specific primers (Table 1) used to measure the relative expression of tight junction proteins (TJPs) were prepared using primer-BLAST software of the National Center for Biotechnology Information (NCBI) (https://www.ncbi.nlm.nih.gov/tools/primer-blast/).
The testis was fixed in 10% neutral-buffered formalin (Sigma-Aldrich). Thereafter, fixed tissues were dehydrated in serial ethanol solutions (50%, 70%, 80%, 90%, and 100%) respectively, and cleared in xylene. Afterward, tissues were embedded in paraffin which was sectioned to 5 μm thickness. Subsequently, sections were deparaffinized from the series of xylene and rehydrated through serial ethanol (100%, 90%, 80%, and 70%), respectively. The tissues were then stained with hematoxylin for 5 min. Finally, the tissue sections were washed in DW and were counterstained with eosin for 15 min. The mounting solution (Sigma-Aldrich) was used to mount the tissue sections for further observation under light microscope.
All experimental data were expressed as mean ± S.E.M. and analyzed using one-way ANOVA on the GraphPad PRISM® (GraphPad Software, San Diego, CA, USA). A completely randomized design was applied, and Tukey’s multiple comparison test was performed to compare the values of individual treatments. The results were considered statistically significant at p-values *p<0.05, **p<0.01, and ***p<0.001.
RESULTS
Significantly higher body weights were observed in both WT-HFD and SIRT1-HFD groups compared to mice not given HFD; WT and SIRT1 groups (Fig. 1A, p<0.05, p<0.01, p<0.001; Fig. 1A). In the comparison of abdominal fat, WT-HFD mice exhibited a significantly higher weight than WT mice and SIRT1 with/without HFD mice (p<0.05; Fig. 1B). There was no significant difference in the liver weight among the groups (Fig. 1C). The weight of the seminal vesicle was significantly higher in the SIRT1-HFD mice (p<0.001; Fig. 1D). However, the testis weight increased more in the WT-HFD mice compared to that of other groups (p<0.05; Fig. 1E). In particular, it was observed that size of the seminal vesicle of SIRT1-HFD mice was morphologically larger than that of mice in other groups (Fig. 1F).
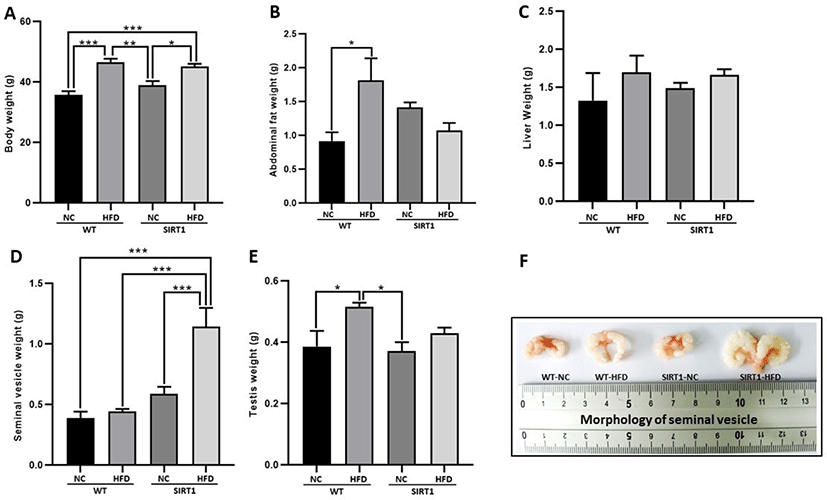
Hepatotoxicity related to the GOT level was higher in the HFD-fed WT and SIRT1-HFD mice than in control NC-diet mice (p<0.05, p<0.01; Fig. 2A). A comparatively higher GPT level was observed in the SIRT1-HFD mice than the levels in the WT with/without HFD or SIRT1 mice without HFD (p<0.01; Fig. 2B). SIRT1-HFD mice showed a significantly higher level of testosterone compared to that of mice in other treatment groups (p<0.05, p<0.01; Fig. 2C). In this experiment, GOT/GPT levels increased in both HFD-fed WT and SIRT1 KO mice (Fig. 2A and B). It was speculated that higher levels of GOT/GPT and testosterone occurred due to the altered lipid metabolism by the SIRT1-deficiency (Fig. 2A and B).
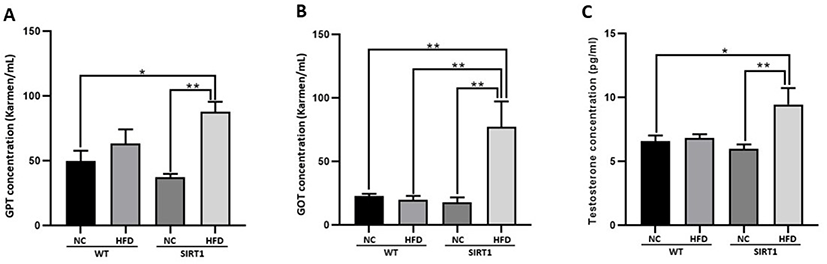
In the serum analysis, the level of IL-1β and TNF-α increased in both WT-HFD and SIRT1-HFD mice (p<0.05, p<0.01, p<0.001; Fig. 3A and C), while lower levels of IL-6 were observed in both WT-HFD and SIRT1-HFD mice (p<0.05; Fig. 3B).
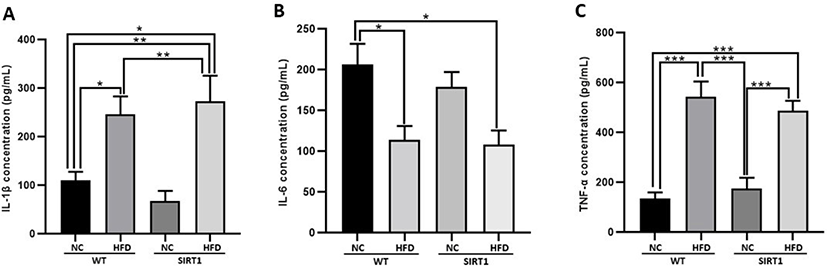
Relative mRNA expressions related to the BTB proteins were analyzed after HFD feeding (Fig. 4). Although there were no statistical differences among groups, the expression levels of occludin and cadherin increased in SIRT1-HFD mice (Fig. 4A and B). TJP1 was highly expressed in SIRT1 mice with or without HFD feeding, but no statistical significance could be drawn (Fig. 4C). However, TJP2 was highly expressed in WT mice, and SIRT1mice both with and without HFD feeding showed low levels of RNA expression (p<0.05, p<0.01; Fig. 4D). HFD-fed WT mice exhibited a remarkably higher expression of claudin 3 than that of the WT or SIRT1 mice (p<0.05, p<0.01; Fig. 4E). The expression of claudin 11 increased in SIRT1 mice with or without HFD feeding, compared to that of the WT mice (p<0.05; Fig. 4F).
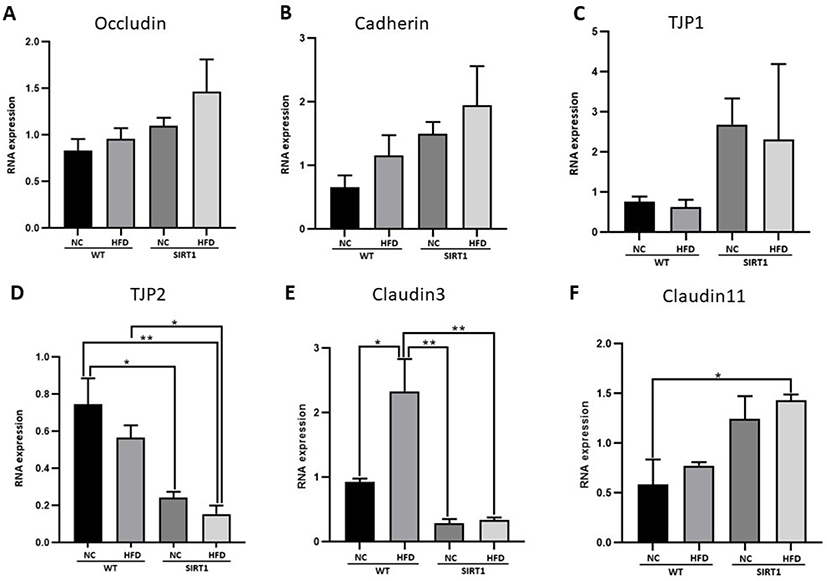
In the histological analysis of the testis, morphological changes were observed in the WT-HFD and SIRT1 mice with/without HFD feeding compared to the WT mice (Fig. 5). Normal sections of the seminiferous tubules were observed in the WT mice (Fig. 5A), while a decreased number of spermatocyte or spermatid, and higher number of defective sperm were detected in the WT-HFD mice (Fig. 5B). As shown in Fig. 5C, degenerative spermatocytes or spermatids were observed in the testis of the SIRT1-NC mice, and such degenerative and defective spermatocytes were highly observed in the testis of SIRT1-HFD mice (Fig. 5D). Sperm motility and concentrations were measured after isolation of the epididymis (Fig. 6). Sperm motility decreased in both WT and SIRT1 mice with HFD feeding (p<0.05, p<0.01; Fig. 6A). The sperm concentration decreased significantly in WT-HFD and SIRT1 mice with/without HFD feeding compared to those of WT-NC mice (p<0.05, p<0.01; Fig. 6B).
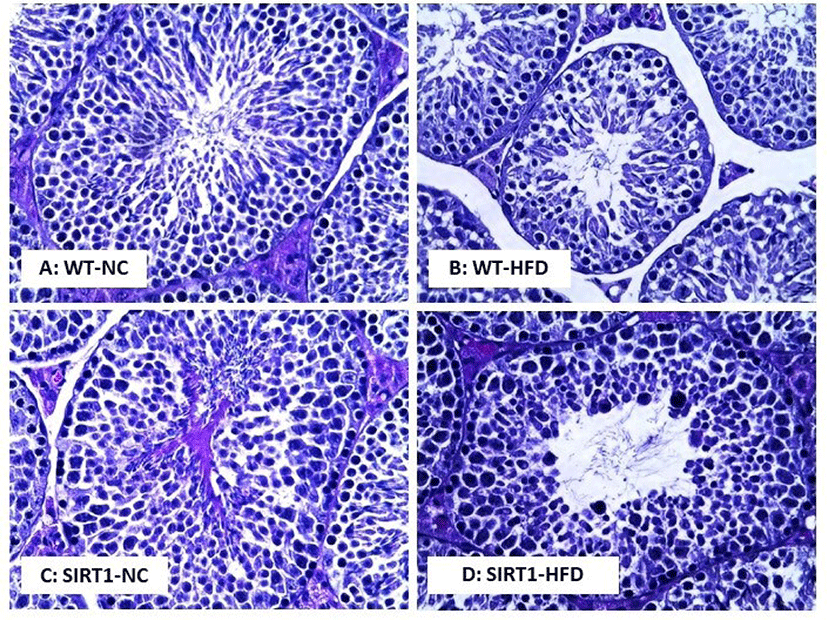
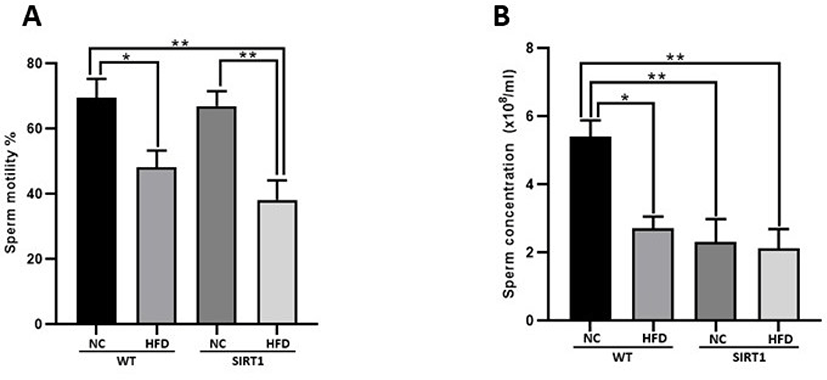
DISCUSSION
SIRT1, one of the members of the sirtuin family plays an important role in the maintenance of metabolic functions and the prevention of obesity [19]. It has been reported earlier that the absence of SIRT1 resulted in increased adiposity that led to metabolic dysfunction [20]. Both body weight and fat mass were highly variable among animals after long-term HFD feeding in this study (Fig. 1), which was consistent with results from previous studies [21, 22]. Specifically, as previously reported, the weight of the seminal vesicle was high in the HFD-treated SIRT1 mice (Fig. 1D), suggesting that steroids and glycolipid metabolism were altered [20].
HFD-fed mice exhibited significantly increased serum levels of GOT and GPT [23], and Abe et al. [24] reported that the elevation of serum GPT levels is related to fat accumulation in the liver cells. In this experiment, GOT/GPT levels increased in both HFD-fed WT and SIRT1 mice (Fig. 2A and B). The liver weights were also slightly higher in the HFD-fed mice, which resulted in fat accumulation and thereby influenced GOT/GPT levels. Men suffering from obesity have been reported to have lower testosterone levels [25]. There is an inverse relationship between obesity and serum testosterone concentrations [26, 27]. Purushotham et al. [20] suggested that HFD elevated the levels of serum testosterone in SIRT1 insufficient mice due to the alteration of the steroid metabolism and defective hepatic androgen inactivation and clearance, which is consistent with our experimental results (Fig. 2).
Earlier studies have shown that obese mice have increased serum IL-1β levels that also increased the caspase-1 activity and IL-1β levels in the adipose tissue [28]. IL-1β selectively damages the insulin-producing β cells in the pancreas [29] leading to impaired β-cell function and reduced insulin production [30]. The expression of IL-6 and TNF-α, two major pro-inflammatory cytokines, was significantly increased in the livers of chronic HFD-fed mice [31]. IL-6 and TNF-α are regulated by the nuclear factor kappa-light-chain-enhancer of activated B cells (NFKB), which is activated by dietary lipids through the toll-like receptor 4, while SIRT1 inhibits NFKB activity. It has also been demonstrated that the overexpression of SIRT1 protects the liver from HFD-induced hepatic damage and maintains normal lipid and glucose metabolism [31]. Therefore, in the absence or insufficiency of SIRT1, lipid accumulation can occur, and cytokine levels are elevated.
The BTB is a structural barrier between the testicular fenestrated capillaries and the interior of the seminiferous tubules. This is of importance in protecting both the spermatogenic cells against blood-borne noxious agents and the seminiferous epithelium from an autoimmune reaction [32]. Also, BTB is constituted by the tight junctions between adjoining Sertoli cells, and occludin, claudin, cadherin, and TJP are essential to its integrity and are often used as biomarkers to assess BTB integrity [33]. The physiology of BTB strongly depends on the steroid activity of mice. Thus, aberrant localization of junctional proteins and their alterations caused due to the changes in steroid activity due to the SIRT1 depletion and HFD consumption [32]. Similarly, in our results, the up-regulation or down-regulation of BTB proteins were observed due to the metabolic imbalance.
SIRT1 is highly expressed in the testis and plays a pivotal role in spermatogenesis [34]. Thus, the depletion of SIRT1 affects the transcription of genes involved in spermatogenesis [35]. Inflammation and oxidative derivatives that are produced from the accumulation of the different components of the HFD, especially cholesterol, affect spermatogenesis in the seminiferous tubules [36]. HFD-induced obesity has been shown to lead to the atrophy of the seminiferous tubules and this can cause a reduction of both spermatogenic series cells and Sertoli cells in rats [37]. Consequently, the structural damage was more prominent in the SIRT1 KO-HFD mice. In other words, aberrant steroid metabolism by SIRT1 depletion elevated the apoptosis of testicular cells and the structural malformation of the testis.
In general, it has been seen that the spermatozoa of HFD-fed male mice showed decreased motility [38, 39]. SIRT1 has been found to play a significant role in the male reproductive system. SIRT1 influences specific functions of the male germ cells during spermatogenesis, especially in the Sertoli and Leydig cells [34]. These effects are caused due to the antioxidant effect of SIRT1 in spermatogenesis. Alam et al. [40] reported oxidative stress and SIRT1 deficiency as the cause of male infertility. The deficiency of SIRT1 decreases the seminal antioxidant defenses in male mice [41]. Accumulation of acetylated microtubule-associated protein 1A/1B-light chain 3 in the spermatid nucleus was caused due to the depletion of SIRT1 in the germ cells, which affects acrosome biogenesis [41]. These changes in spermatogenesis lead to an increased proportion of abnormal spermatozoa and a decrease in motility and sperm concentrations in the SIRT1-deficient. In conclusion, SIRT1 deficiency alters energy and steroid metabolism in mice on HFD, which can lead to imbalances in the production of testosterone and sperm that can lead to male reproductive disorders.