INTRODUCTION
Iron is a mineral that is naturally present in many foods, is added to some food products, and is available as a dietary supplement. The characteristic of iron to stably interconvert between its most common oxidative forms, Fe2+ and Fe3+, has been extensively exploited in biological systems [1–3]. Iron is an essential component of hemoglobin, a red blood cell protein that serves as a carrier of oxygen from the lungs to the tissues, and as an integrated part of important enzyme systems in various tissues [4]. Iron is a component of myoglobin, another oxygen-supplying protein, which regulates muscle metabolism and maintenance of connective tissue [5]. Iron is also required for physical growth, neurological development, cell function, and the synthesis of hormones such as hepcidin [5, 6]. Iron intake recommendation is provided in the Dietary Reference Intakes developed by the Food and Nutrition Board (FNB) at the Institute of Medicine of the National Academy of Sciences. According to the USA Institute of Medicine [7], the Recommended Dietary allowances of iron for women and men aged 19 and above are 18 and 8 mg/day, respectively.
Iron deficiency is a common nutritional disorder in many countries, especially among young children, women of reproductive age, and pregnant women. People with iron deficiency usually have other nutrient deficiencies because iron deficiency is associated with poor diet, poor absorption, and blood loss. In developing countries, iron deficiency often results from intestinal parasites and blood loss [5]. The World Health Organization (WHO) estimates that approximately half of the 1.62 billion cases of anemia worldwide are because of iron deficiency [8]. Iron deficiency is the most common cause of anemia, but deficiencies of other micronutrients (e.g., folic acid and vitamin B12) and other factors (e.g., chronic infection and inflammation) can cause different forms of anemia or contribute to the severity of anemia. Meanwhile, excessive iron intake (more than 20 mg/kg) from supplements or medicines can lead to gastritis, constipation, nausea, stomachache, vomiting, and faintness, especially if not taken with food [5, 7]. Zinc absorption and plasma zinc concentrations can be reduced by taking iron supplements [5, 9, 10]. In severe cases, excessive iron can lead to multisystem organ failure, coma, convulsions, and even death [11, 12].
Food supplementation refers to the addition of essential trace elements and vitamins to food, to provide a public health benefits while improving nutritional quality and minimizing health risks. This is a relatively simple and efficient method to prevent mineral deficiencies. Ferrous and ferric iron salts, such as ferrous sulfate, ferrous gluconate, ferric citrate, and ferric sulfate are frequently used forms of iron in supplements [6, 12]. However, mineral fortification in foods is challenging because it often causes unexpected changes during preparation and storage, low bioavailability, and high coast [13]. Application of nanotechnology can overcome these shortcomings in food fortification, for example, by using colloidal techniques, surface coating, emulsification, etc [14]. SUNACTIVE Fe-P80 is a new type of iron supplement that applies nanotechnology for the purpose of overcoming the disadvantages of food supplements. In addition to the benefits of nanotechnology in food preservation and fortification, some researchers have highlighted significant safety concerns because it is unknown at what concentration or size nanoscopic materials may start to demonstrate novel toxicological features. The safety of nano-foods and dietary supplements must be evaluated through repeated oral dosage toxicities in in vivo investigations.
This study was carried out to investigate the no-observed-adverse-effect level (NOAEL) and target organs of SUNACTIVE Fe-P80. SUNACTIVE Fe-P80 was orally administered to male and female Sprague-Dawley (SD) rats for 28 days, and qualitative and quantitative tests were performed. This study was conducted in accordance with the test guidelines of the Korean Ministry of Food and Drug Safety [15] and the Organization for Economic Cooperation and Development guidelines [16] for the testing of chemicals under modern Good Laboratory Practice (GLP) regulations.
MATERIALS AND METHODS
A total of 70 SD rats (35 males and 35 females) approximately 5 weeks old and within weight range of 10 g for both sexes were received from a specific pathogen-free colony at the Orient Bio (Gapyeong, Korea). Animal room temperature and humidity controls were generally within 20℃ to 26℃ and 40% to 70% respectively. Artificial lighting was controlled to give 12 hours continuous light and 12 hours continuous dark per 24 hours with a luminous intensity of 150–300 Lux and 10–20 air changes per hour. The rats were placed at random in suspended cages with wire mesh floors with stainless steel wire cage (W310 × L500 × H200 mm), according to sex, so that each cage contained 5 rats of the same sex. After an acclimatization period of 7 days, each animal was weighed and the required number of animals was selected by discarding those animals furthest from the mean bodyweight. The remaining animals were then randomly assigned to cages, stratified by bodyweight, in such a way that the initial cage means were approximately equal. The appropriate numbers of cages were then allocated to each treatment group. At the commencement of the study, the range of bodyweight variation in the animals used did not exceed 20% of the appropriate mean values. Later, the group size was decreased to two animals per cage during the administration and observation periods. The proper laboratory animal pellet meal (Labdiet 5L79, PMI Nutrition International, St. Louis, MO, USA), which was acquired from Orient Bio, was made available to the animals at all times. Underground water was provided freely in the form of a water bottle after being sterilized with UV light and put through ultrafiltration. The protocols utilized in this animal study were approved by Korea Testing and Research Institute’s Institutional Animal Care and Use Committee, and the animals were cared for in accordance with the Guide for the Care and Use of Laboratory Animals (Approved number: IAC2018-112) [17].
Before usage, SUNACTIVE Fe-P80 (26.6% ferric pyrophosphate, 54.7% dextrin, 7.3% sodium chloride, 5.3% glycerol esters of fatty acids, 4.8% water, and 1.3% enzymatically hydrolyzed lecithin) was kept at room temperature (15℃ to 25℃) which was provided by Taiyo Kagaku (Yokkaichi, Japan). Orally administered sterile distilled water (Daihan Pharm, Seoul, Korea), which was stored under refrigeration (0℃–10℃) after opening, was used as the vehicle control. SUNACTIVE Fe-P80 for oral administration was prepared by suspending it in sterile distilled water at the appropriate dose for each assigned group. SUNACTIVE Fe-P80 was analyzed by XRD (Bruker AXS D2 Phaser, Bruker, Billerica, MA, USA) to obtain an amorphous phase. It was then measured using a dynamic light scattering instrument (ELSZ-1000, Otsuka, Tokyo, Japan) to obtain 240 nm hydrodynamic radii. It showed a sphere-like morphology at low magnification, which seems to be attributed to the organic moiety, such as dextrin and glycerides. Primary particle size was shown in magnified scanning electron microscopy (Quanta 250 FEG, FEI Company, Hilsboro, OR, USA) pictures to be 40 ± 10 nm.
During the administration period, the concentration and/or homogeneity of SUNACTIVE Fe-P80 at 50, 100, and 200 mg/mL dosages in sterile distilled water were examined by ICP-AES (HORIBA JOBIN YVON ULTIMA2, HORIBA, Longjumeau, France) and found to be within the permitted limits (concentration nominal value ± 10%; homogeneity within 5%).
This study was conducted according to OECD testing guidelines 407 [16]. The dose levels were selected based on the results from a previous 7-day study previously performed in the SD rat where administration up to the highest dose of 2,000 mg/kg/day resulted in no mortalities or clinical signs, no treatment effect on body weight or food consumption, no effect on organ weight and no macroscopic findings in either sex (data not shown). Based on the results of a preliminary study, a high dose of 2,000 mg/kg/day was administered. Doses of 1,000 and 500 mg/kg/day were set as the middle and low doses, respectively, using a common ratio of 2. The vehicle control group was orally administered sterile water only. Each group consisted of 5 (low and middle dose groups) or 10 (vehicle control and high-dose groups) rats of each sex. In order to monitor the reversibility, persistence, or delayed development of toxic effects for 14 days after treatment, there were recovery groups of five animals per sex in both the high-dose and control dose groups.
The oral method was chosen because that is how supplements are typically given to people. Using a sonde and syringe, the prepared test material was immediately injected into the stomach. The most current body weight data were utilized to determine the dosing volume, which was 10 mL/kg/day. For 28 days, the test material was given every day.
Animals were checked for moribundity and mortality twice daily. Animals were observed for clinical signs at least once daily for all animals during the study. Detailed physical examinations were performed at least weekly during the treatment and recovery periods. The nature, onset, severity, duration and recovery of clinical signs were recorded. Cages and cage trays were inspected daily for evidence of ill health such as blood or loose feces. Each animal was weighed three times during the acclimatization period, on the first day of test item administration, then at least weekly throughout the treatment and recovery periods. Additionally, diet fasted animals were weighed before scheduled necropsy (terminal body weight). The weight of food supplied and of that remaining at the end of the food consumption period was recorded weekly for all animals during the treatment and recovery periods. From these records the mean daily consumption was calculated.
During the acclimatization period, all animals were subjected to an ophthalmic examination. After instillation of an atropinic agent (Mydriatics, Ocuhomapin, Samil Pharm, Seoul, Korea) each eye was examined by means of an indirect ophthalmoscope (Genesis, Kowa, Nagoya, Japan). The anterior eye structures were examined, as well as the eye when the pupil had dilated. During day 28 of the treatment period, all surviving animals from control and high dose group were re-examined.
On study Day 27 (for animals of the main study phase) and on recovery Day 13 (for animals of the recovery phase), in the morning, overnight urine samples were collected from all surviving animals in all groups. Five animals from each group were sampled on each day. Food and water were not accessible during urine collection. Urine samples were weighed to determine urinary volume.
The bilirubin, glucose, specific gravity, ketone bodies, pH, occult blood, protein, nitrite, urobilinogen, and white blood cell count were assayed using an automatic analyzer (Clini-Tek 500, Ames Division, Miles Laboratory, Elkhart, IN, USA).
Urinary refractive index was measured using a RFM320 refractometer (Bioblock Scientific, Illkirch, France). Microscopic examination of the urinary sediment was performed after centrifugation (425 RCF, MF300, Hanil, Gimpo, Korea) of the urine. The presence of red blood cells, white blood cells, epithelial cells, bacteria, casts and crystals was graded.
On the day of scheduled sacrifice (Study Day 28 for animals of the main study and on Recovery Day 14 for animals of the recovery phase), blood samples were taken from the vena cava of all surviving animals in all groups. Animals were diet fasted overnight prior to bleeding and anesthetized by inhalation of Isoflurane (Virbac, Carros, France). Blood was collected on EDTA for hematology (approximately 0.5 mL) and on sodium citrate for coagulation parameters (approximately 0.9 mL). Hematology parameters were measured using an automatic hematology analyzer Coulter counter (ADVIA 120E, Siemens, PA, USA): WBC, WBC differential counts (neutrophils, lymphocytes, monocytes, eosinophils, and basophils), reticulocytes, hematocrit, mean corpuscular hemoglobin (MCH) concentration, hemoglobin concentration, mean corpuscular volume, platelet count, red blood cell count, and MCH. In order to measure the time it takes for blood to clot, plasma was taken and centrifuged (735 RCF, Microcentrifuges 5402, Eppendorf, Hamburg, Germany) for 10 min. The nephelometric analysis method was used to measure the prothrombin time and activated partial thromboplastin time in seconds using a coagulation time analyzer (ACL 7000, Instrumentation Laboratory, Bedford, MA, USA).
For serum clinical chemistry, blood was drawn through a clot activator (about 1.1 mL) and let to sit at room temperature for 90 minutes. Then, it was centrifuged (5,000 × g, 10 min), and the serum was separated for hematological analysis. Parameters were measured using a serum biochemistry analyzer (TBA-120FR, TOSHIBA, Tokyo, Japan): alanine aminotransferase, aspartate aminotransferase, alkaline phosphatase, total bile acid, total bilirubin, creatine phosphokinase, glucose, triglyceride, total cholesterol, albumin, albumin/globulin ratio, total protein, creatinine, blood urea nitrogen, gamma-glutamyl transpeptidase, inorganic phosphorus, ferritin, sodium ion, chloride ions, potassium ion, and calcium.
On study Days 28 of the dosing phase for the 28-day treatment phase, on Days 14 of the recovery phase for the reversibility sacrifice, all surviving animals from all groups were sacrificed by exsanguination under deep anesthesia (inhalation of Isoflurane). Animals were diet fasted overnight prior to sacrifice. All animals were necropsied. All significant organs, tissues, and body cavities were examined during the necropsy. Macroscopic abnormalities were recorded, sampled and examined microscopically.
The brain, heart, kidneys, spleen, epididymides, testes, prostate gland (prostate and seminal vesicles with coagulating glands), ovaries, adrenal glands, thymus, uterus, and liver were weighed at necropsy for all animals scheduled for euthanasia. Organ pairs were weighed independently. Based on the terminal body weight, relative organ weight was determined.
All of the major organs and tissues of each animal were macroscopically inspected after a thorough necropsy. Histopathological evaluation of all organs and tissues from the control and high-dose groups was performed. The heart, thymus, brain, lungs, spleen, kidneys, aorta, trachea, nasal cavity, liver, pituitary, parathyroid glands, thyroid, adrenal glands, tongue, stomach, esophagus, salivary glands, jejunum, cecum, duodenum, colon, ileum, rectum, urinary bladder, uterus, pancreas, ovaries, seminal vesicles, mammary glands, vagina, epididymides, testes, prostate, skin, cervical and mesenteric lymph nodes, bulbourethral glands, trigeminal nerve, sternum and femur including bone, marrow, spinal cord (cervical, thoracic, and lumbar cord), sciatic nerve, Harderian glands, eyes, thigh muscle, and gross abnormalities were excised.
Except for the testicles, which were fixed in Bouin solution, and the eyes, including the optic nerve and Harderian glands, which were fixed in Davidson’s fixative, all of the aforementioned organs and tissues were fixed in 10% buffered formalin. All of the above samples were embedded in paraffin wax, as previously described [18, 19]. Histological sections, stained with hematoxylin and eosin, were prepared from all organs and tissue samples from all the animals in the control and high dose groups and from animals which died or were sacrificed before scheduled sacrifice/from all animals. Specimens were then processed for histopathology at the Preclinical Research Center, Korea Testing & Research Institute (Hwasun, Korea), which is a GLP institute approved by the MFDS.
Male and female statistical analyses were carried out separately. Numerical data are presented as means with S.D. for each group. Food consumption, body weight, hematology, urine volume, organ weights and serum biochemistry were assumed to be normally distributed and analyzed by one-way analysis of variance and Student’s t-test for recovery group comparisons. Using Levene’s test, the homogeneity presumption was examined. If the assumption of homogeneity of variance was not met, Dunnett’s T3 test was used as the post-hoc test [20]. Urinalysis data were rank-transformed and analyzed using the non-parametric Kruskal-Wallis H-test [21]. If a statistically significant difference was observed between the groups, the Mann-Whitney U-test was used to identify the groups that were significantly different from the vehicle control group [22].
For categorical data, including estrous cycle, clinical signs, necropsy, and histopathological findings, the proportion of animals was analyzed using Fisher’s exact probability test [23] for each treated group versus the control group. SPSS version 19.0 was used for all statistical analyses (SPSS, Chicago, IL, USA). The significance of the differences between the control and treatment groups was estimated at probability levels of 1% and 5%.
RESULTS
Throughout the study period, there were no treatment-related clinical signs, deaths, or moribund sacrifices (data not shown). As shown in Table 1, there were no significant differences in body weight between the vehicle control and the treatment groups during the experimental period in either sex. Changes in food consumption in SUNACTIVE Fe-P80-treated rats are summarized in Table 2. Results showed that food consumption significantly decreased on day 8 of the test in the male 500 and 1,000 mg/kg/day groups compared to the vehicle control group (p<0.01). In females, however, no significant differences were observed between the vehicle control and treatment groups.
No treatment-related changes were observed in urinalysis- and ophthalmic examination-related parameters at the end of the experimental period (data not shown).
Results of the hematological examination are shown in Tables 3 and 4. MCH for males in the 2,000 mg/kg/day group was significantly increased (p<0.05) compared with that for the males in the vehicle control group. Neutrophil count was significantly increased (p<0.05) in the female 1,000 mg/kg/day group, compared to the vehicle control group. Significant changes in the hematological parameters observed in the males and females of the treatment groups were not observed at the end of the recovery period (data not shown).
Serum biochemistry results are shown in Tables 5 and 6. Triglyceride levels in males in the 1,000 mg/kg/day group were significantly decreased (p<0.05) compared with those in males in the vehicle control group. In females, however, no significant differences were observed between the vehicle control and treatment groups. At the end of the recovery period, no significant differences in serum biochemical parameters were observed between the vehicle control and highest dose groups (data not shown).
AST, aspartate aminotransferase; ALT, alanine aminotransferase; ALP, alkaline phosphatase; CPK, creatine phosphokinase; TBIL, total bilirubin; TBA, total bile acid; TCHO, total cholesterol; TP, total protein; A/G, albumin/globulin; BUN, blood urea nitrogen; IP, inorganic phosphorus; GGT, gamma glutamyl transpeptidase; Fe-L, ferritin.
AST, aspartate aminotransferase; ALT, alanine aminotransferase; ALP, alkaline phosphatase; CPK, creatine phosphokinase; TBIL, total bilirubin; TBA, total bile acid; TCHO, total cholesterol; TP, total protein; A/G, albumin/globulin; BUN, blood urea nitrogen; IP, inorganic phosphorus; GGT, gamma glutamyl transpeptidase; Fe-L, ferritin.
At the planned necropsy, no treated animals displayed any gross findings attributable to the treatment (data not shown). The absolute and relative organ weights of the SUNACTIVE Fe-P80-treated male and female rats are presented in Tables 7 and 8. No treatment-related changes were observed in absolute or relative organ weights at the end of the experimental period between the groups.
The results of the histopathological examination are presented in Table 9 and Fig. 1. Inflammatory cell infiltration in the liver was observed in one and two males in the vehicle control and 2,000 mg/kg/day groups, respectively, and focal necrosis in the liver was observed in one male in the vehicle control group. Interstitial inflammatory cell infiltration in the kidney was observed in one male in the 2,000 mg/kg/day group. Tubular dilation in the kidney was observed in one female in the 2,000 mg/kg/day group, and tubular cast in the kidney was observed in one female in the vehicle control group. Vacuolation in the pituitary glands was observed in one male in the 2,000 mg/kg/day group. Extramedullary hematopoiesis in the spleen was observed in one male each in the vehicle control and 2,000 mg/kg/day groups. Interstitial inflammatory cell infiltration in the pancreas was observed in one male in the 2,000 mg/kg/day group. At the end of the recovery period, no histopathological alterations in the liver, kidney, pituitary glands, spleen, or pancreas were observed in the highest dose group in either sex (data not shown).
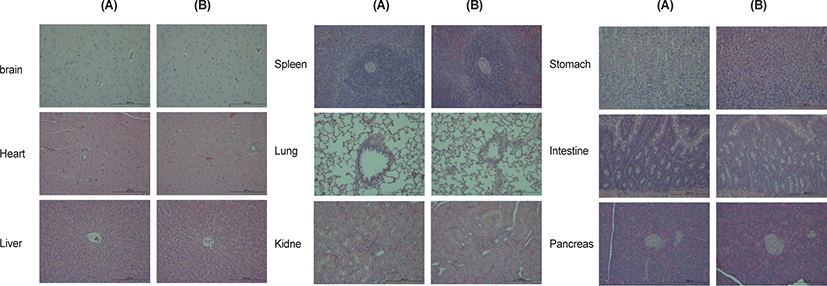
DISCUSSION
In order to establish the NOAEL and target organs, this study examined the possible subchronic toxicity of SUNACTIVE Fe-P80 in rats after a 28-day repeated oral treatment. The findings of this investigation demonstrated that repeated oral administration of SUNACTIVE Fe-P80 to rats for 28 days at doses of less than 2,000 mg/kg/day did not result in any treatment-related side effects.
Our results showed that 28-day repeated oral administration of SUNACTIVE Fe-P80 did not result in any clinical signs of toxicity or death at any dose tested in either sex. No abnormal changes in body weight or food consumption were observed during the treatment or recovery period. The significant decreases in food consumption observed in the male 500 and 1,000 mg/kg/day groups were not considered to be toxicologically significant because they were not dose-related, and were unaccompanied by correlated changes in other parameters.
The significant changes in hematological and serum biochemical parameters (MCH, neutrophils, and triglycerides) observed in the treatment groups were also considered incidental since the findings lacked a dose-response relationship and were not accompanied by other relevant findings. Moreover, such changes were within an acceptable historical range [24, 25].
No abnormal changes in necropsy findings or absolute and relative organ weights were observed at any dose tested. Although some histopathological alterations, including inflammatory cell infiltration in the liver, interstitial inflammatory cell infiltration, and tubular dilation in the kidney, vacuolation in the pituitary glands, extramedullary hematopoiesis in the spleen, and interstitial inflammatory cell infiltration in the pancreas, were observed in the 2,000 mg/kg/day group for both sexes, they were considered to be incidental findings, as their incidence and severity were similar to those in their respective control groups. Moreover, these findings are commonly observed in normal control rats [19, 26–28].
Serum iron and ferritin are the most commonly used hematological measures to assess the effect of iron supplementation. Unlike serum iron, serum ferritin concentration does not fluctuate from day to day. Because of its relatively high stability and solubility, as well as its direct proportionality to body iron stores in normal persons, serum ferritin is the most commonly used indicator of total body iron storage. In this study, serum ferritin levels did not increase with a dose-response relationship. However, the animals were not in iron deficiency, and iron absorbed by gavage is homeostatic in the body, and the rest is send out of the body. So, SUNACTIVE Fe-P80 can be expected to be safe even when exposed due to increased capacity.
To date, the toxicity of many micronutrients such as iron is well understood, but the data available on the concentration or size will begin to exhibit new toxicological properties because the nanoscopic dimensions are limited. Previous studies have shown that nanoparticles may cause several cytotoxic and genotoxic effects owing to their unique properties, such as a large surface area-to-mass ratio [29, 30]. Nanoparticles are internalized by cells, resulting in genetic mutations through interactions with cellular organelles. The causes of genetic toxicity induced by nanomaterials are either direct interactions with genetic materials (DNA and RNA) or indirect damage by reactive oxygen species (ROS) [31, 32]. ROS generation by nanomaterials has been observed both in vitro and in vivo [33–37]. Recently, it was also reported that TiO2 nanoparticles can induce tumor-like changes human cells exposed to them [38–40]. In contrast, it was found that toxicity may be effectively prevented through surface chemical changes of nanoparticles [41–44]. The surface chemistry and surface charge of nanoparticles play important roles in toxicity and corresponding safety assessments [45]. For example, polysaccharide coatings have been used to promote biocompatibility and better dispersion of solutions [46, 47]. With regard to the surface charge, positively charged Au nanoparticles caused greater toxicity than negatively charged particles [48]. Further, Al2O3 nanoparticles were less toxic than metallic Al nanoparticles, suggesting that surface oxide formation may also change bio-interaction [49]. In addition, ultra-small (~4–5 nm) nanodiamonds, various functionalized carbon nanotubes, and cerium nanoparticles have not shown any distinct toxic effects on cells in culture [50–55]. The results of this study showed that 28-day repeated oral administration of SUNACTIVE Fe-P80 in rats did not result in any treatment-related adverse effects up to a dose level of 2,000 mg/kg/day, which is considered an acceptable upper limit dose according to ICH guidelines [56]. The results of this study clearly show that a 28-day repeated oral administration of SUNACTIVE Fe-P80, which applies nanotechnology, does not cause any toxic effects in rats.
In conclusion, the study showed that 28-day repeated oral administration of SUNACTIVE Fe-P80 had no significant adverse effects in rats. Under the present experimental conditions, the NOAEL was ≥ 2,000 mg/kg/day in both sexes, and no target organs were identified. Thus, the results obtained from this study suggest that SUNACTIVE Fe-P80 is relatively safe, as no treatment-related adverse effects were observed following a subchronic toxicity experiment. Overall, our results are expected to provide useful information on the general toxic effects of SUNACTIVE Fe-P80 via repeated oral exposure, which can aid in risk assessment.