Introduction
The rapid economic and industrial growths have resulted in the development of numerous tools to generate a convenient lifestyle. However, this has resulted in causing environmental pollution, including heavy metal contamination. Especially, different industrial fields such as cosmetics, medicinal, agricultural and electronics, use heavy metals as their primary input [1]. Anthropogenic activities, including excessive mining activities of minerals and improper waste handling, have promoted increased environmental pollution [2, 3]. Although lead (Pb) is a known naturally existing heavy metal, its abundance in the natural environment has increased due to the anthropogenic activities of humans [1].
Human exposure to Pb generally occurs through atmospheric dust, paints, automobile exhausts, and long-term ingestion of contaminated food and water [4]. Pb is also a known neurotoxin and carcinogen in humans, and is responsible for central nervous system damage, affective disturbances and neurocognitive disturbances, as well as being the causative agent of malignancies by damaging the DNA through impairing the DNA repair mechanism or replacing zinc in the DNA inhibition protein [5]. Mechanisms that lead to the carcinogenic effects are chronic neuropathy, mitogenesis, oxidative damage, and alterations in gene transcription [6].
Using planarians in toxicity studies became popular due to their specific inhabited characteristics, such as having the ability to reproduce both asexually and sexually, a short lifecycle, and simple body structure with regeneration abilities [7, 8]. Also, their structural and molecular similarities to the mammal brain increases their experimental value as an animal model [9]. Other beneficial characteristics of planarians in laboratory experiments include cost effectiveness (inexpensive), easy to culture, easy maintenance, less space requirement as compared to other species, and sensitivity towards toxicants [10]. The present study was therefore undertaken to investigate alterations of the typical characteristics in planarians exposed to Pb.
Materials and Methods
The animal model used comprised approximately 1.0 cm long planarians (Dugesia japonica), acclimated in freshwater at 18°C. Animals were fed finely homogenized beef liver twice a week. Uniform metabolic state within the planarian was created by starving them for one week prior to the experiment. Lead (II) nitrate [Pb(NO3)2] was purchased from Sigma-Aldrich (Seoul, Korea). Chemicals were dissolved in DW and used in the experiment.
Motility of the planarian was measured by evaluating the swimming ability in its natural state. Briefly, petri dishes (p-dish) filled with the treatment solutions were placed on a graph paper divided into a grid of 0.5 cm intervals. Motility was evaluated by counting the number of grid lines that each planarian passed. Simultaneously, the planarian seizure-like behaviors were also observed, and classified into four categories: c-like, head-bop, snake-like, and screw-like behavior [11, 12]. Planarians were incubated in 10 mL experimental solutions containing 10–400 mg/L Pb, for 1–5 hr, and were subsequently evaluated for motility and seizure-like behaviors (number of grid lines crossed or re-crossed by each planarian) within 5 min of termination of the incubation period.
Planarians were paralyzed by chilling on ice, after which the stretched bodies were cut using an ethanol-sterilized blade. The head region on the auricular grooves was decapitated, and rest of the body was incubated in water containing Pb at 18°C, until emergence of eye extrusion. The imaging process of the regeneration fragments was conducted for 14 days, using a stereo-microscope (S8APO, Leica, Wetzlar, Germany).
Immunofluorescence staining was conducted as previously described, but with slight modification [13]. Briefly, excess mucus was removed by subjecting the regenerating planarians to 2% HCl diluted in DW for 5 min. The planarians were subsequently fixed in Carnoy’s solution (ethanol:chloroform:acetic acid = 6:3:1) for 3 hr at RT, followed by rinsing with 100% methanol, and bleached overnight under a light emitting diode (LED) lamp at RT, in 6% H2O2 diluted in methanol. The bleached samples were rehydrated through a graded methanol series (75%, 50%, and 25%) diluted with phosphate buffer saline (PBS), for 15 min at each concentration. Samples were then rinsed in PBS containing 0.3% Triton X-100 (PBST), blocked in PBST containing 0.25% BSA (PBSTB) for 2 hr, and subsequently incubated with rabbit polyclonal anti-arrestin antibody (LAgen Laboatories, 1:5,000 dilution) in PBSTB, overnight at 4°C. Probed samples were washed 3 times with PBSTB for 2 hr, followed by incubation with goat rabbit anti-Alex488 IgG antibody in PBSTB (Invitrogen, 1:2,000 dilution) and 0.5 μg/ml 4’6’-diamidino-2-phenylindole (DAPI), overnight at 4°C in the dark. After incubation, samples were washed with PBST, and finally mounted on slides and observed using a fluorescence microscope (Nikon Eclipse Ci, Nikon, Seoul, Korea).
Results
As shown in Fig. 1, planarians were incubated in water containing varying concentrations of Pb for 1–5 hr. Motility was observed to significantly decrease in a dose-dependent manner after 1 h incubation (p<0.05, p<0.01, & p<0.001; Fig. 1A). After further incubation from 3–5 hr, similar decreasing motility patterns were observed, as seen with 1 hr incubation (p<0.05, p<0.01, & p<0.001; Fig. 1B & C). On the other hand, there was no statistical significance of motility in the planarians exposed to 10 and 50 mg/L Pb at 3 and 5 hr of incubation, compared to motility at the same concentrations after 1 hr incubation. Presumably, accumulation of Pb in the body increases as the incubation time increases, and the differences in motility between treatments is expected to decrease (Fig. 1). Exposure to Pb also induced seizure-like behaviors (Fig. 2). Significantly higher numbers of c-like behavior were observed in planarian exposed to higher concentrations of Pb (100–400 mg/L) during 1–5 hr incubation (p<0.05, p<0.01, & p<0.001; Fig. 2A). Also, frequencies of head-bop behaviors were increased in planarians incubated with Pb for 1–5 hr incubations, as compared to planarians without Pb (p<0.05; Fig. 2B). The snake-like and screw-like behaviors were only observed when planarians were incubated in the presence of 400 mg/L Pb (p<0.05; Fig. 2C & D). These results imply that exposing planarians to Pb alters their normal behavioral patterns.
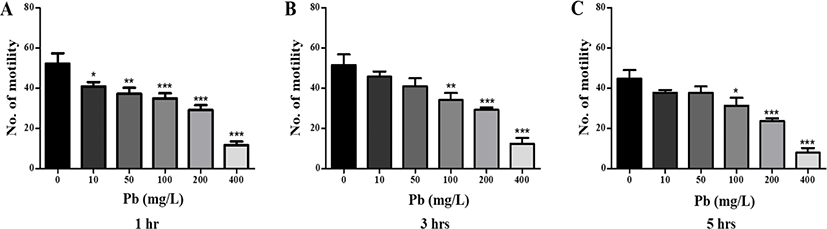
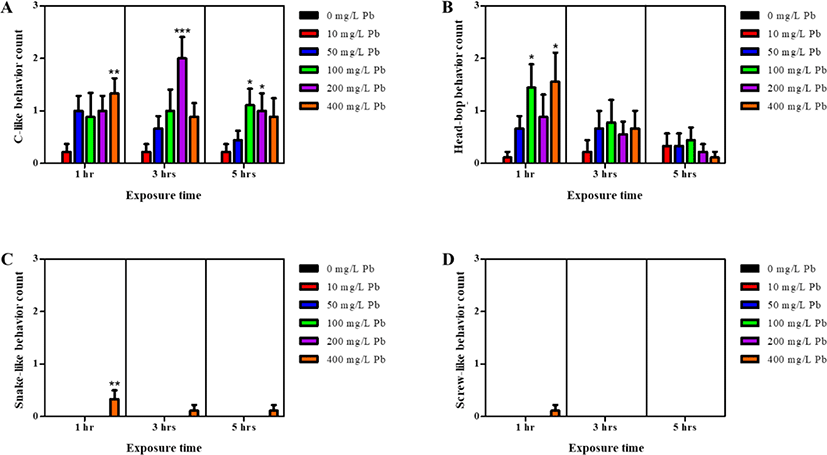
For determining regeneration from amputated fragments, the head region on the auricular grooves was decapitated, and rest of the body was incubated in water, in the absence or presence of Pb (Fig. 3). Incubation was continued until the emergence of eye extrusion. As shown in Fig. 3A, regeneration was determined by calculating the indicated scores of 0, 0.5 and 1 according to burst, regeneration and completion of regeneration, respectively. The control group (without Pb) was spotted with the eye on 5 day, while extrusion of eye spots was delayed in planarians exposed to 200 mg/L Pb (Fig. 3B), whereas no eye spots were formed in planarians exposed to 300 mg/L Pb (Fig. 3B). Although planarians incubated in the absence of Pb or presence of 200 mg/L Pb showed 90%–100% survival rate until 14 days, planarians exposed to 300 mg/L Pb showed 40% survival on day 5, but 100% mortality on day 8 (Fig. 3C).
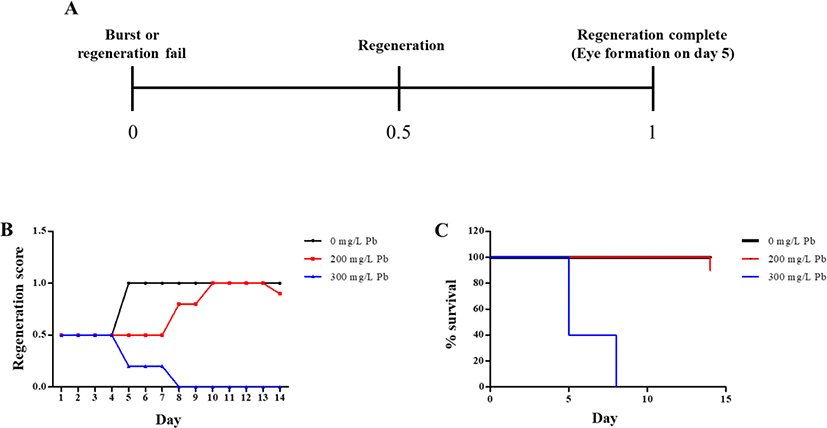
During incubation of the fragments, eye formation was observed through a stereo-microscope (Fig. 4). Eye formation appeared in planarians cultured in water in the absence of Pb on day 5 (Fig. 4A). The 200 mg/L Pb exposed group was unable to form eye spots on the same day, but showed delayed eye formation on day 8 (Fig. 4B). However, eye formation was completed in all planarians cultured with or without Pb on day 14 (Fig. 4A & B). Immunohistochemistry using anti-arrestin antibody was performed to observe the development of optic nerves derived from the amputated fragments in each treatment (Fig. 5A & D). Clear eye spots (blue; DNA) with optic nerves (green; arrestin) were observed in planarian cultured in the absence of Pb on day 5 (Fig. 5B & C), while optic nerves were not completely formed in planarian incubated with 200 mg/L Pb (Fig. 5E & F).
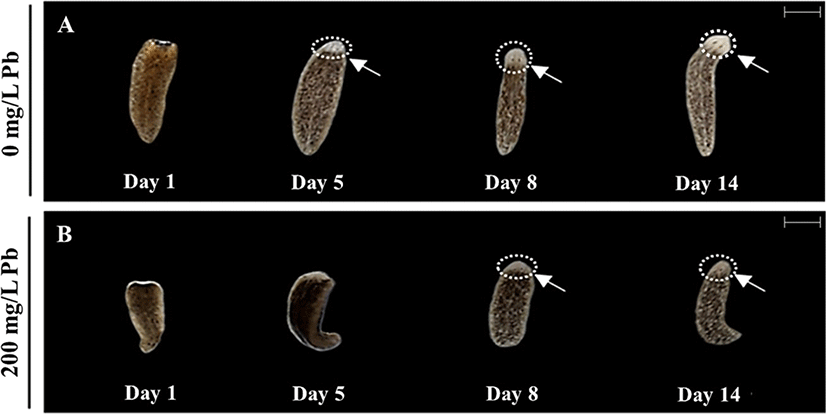
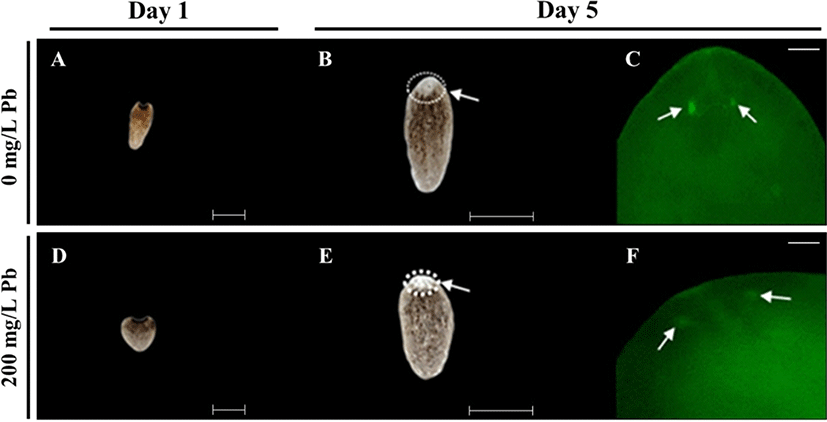
Discussion
Heavy metals are known as naturally existing substances with high atomic weight which are released by naturally occurring events. Along with the rapid development of almost all industries (agricultural, pharmaceutical, technological etc.), there has been a concurrent increase in the environmental pollution. Anthropogenic activities of humans contribute greatly to environmental pollution [14]. Among the heavy metals, Pb is one of the most commonly used metals in numerous industries, and due to the mishandling of industrial waste, these metals are easily introduced into the natural environment [15, 16]. Pb has the ability to induce neurotoxicity in humans. Common routes for Pb exposure are air, food and water. Additionally, most the canned food packages are made by incorporating Pb, thereby making it a major health risk [4, 17].
Accumulating Pb in the body results in poisoning of the nervous system, hematopoietic system, and kidneys [18]. Acute Pb poisoning causes abdominal pain, confusion and headache [19]. Moreover, in severe intoxications, it can cause coma, seizures and even death of the patient. Pb also induces neurophysiological and neuropsychological defects based on the level of accumulation in the body. Moreover, Pb exposure is also reported to cause anxiety, depression, as well as decreasing levels of memory, processing speed, visuospatial skills and motor skills [20].
Results of the present study determined decreased planarian motility and increased seizure-like behaviors with increments in the Pb concentration (Fig. 1 & 2). Additionally, in longer the exposure time to Pb, the less differences in the number of motility and seizure-like behavior were observed among treatments. This is presumed to cause a slowdown in motility nerves by gradually accumulating Pb in the body of planarians. Inability to regenerate was also observed during the study period (Fig. 3–5). Normally, Pb exerts its toxic effect by damaging the DNA, and has the ability to inhibit the DNA repair machinery and replace zinc in the DNA binding proteins [6]. Several studies report that exposing planarians to Pb damages the regeneration abilities, feeding behaviors, and decreases the mobility [21, 22], which is similar to results obtained in the current study. There are numerous other studies which report the toxicity of Pb in invertebrates who live either in the water or soil. A study of Pb toxicity on the oyster (Crassostrea gigas) stated that protein metabolism is disturbed by inducing endoplasmic reticulum (ER) stress. Also, it stated that exposure to Pb increases the arachidonic acids, which indicate possible alterations in the unsaturated fatty acid composition and disturbs the cellular membrane function [23]. Another study in the nematode (Caenorhabditis elegans) stated that a combined effect of Pb with manganese (Mn) causes synergistic lethal toxicity for the animals, and individual exposure results in adverse effects on the reproduction, lifespan, stress response and neurotoxicity [24]. Toxicity evaluation in freshwater bivalves showed that Pb exposure led to alterations in the behavioral patterns such as movements and foot mobilization. The same study also reported that Pb exposure elevated lipid peroxidation and altered the catalase activity, resulting in oxidative stress in the cells [25].
We conclude that Pb has the ability to induce toxic effects in planarians, stimulate seizure-like behaviors, and affect the eye regeneration. Taken together, results of this study clearly indicate the health risks associated with lead exposure in living organisms, including humans.