Introduction
Gastrointestinal damage induced by irradiation is the most common side effect observed in patients undergoing radiation therapy [1, 2]. These patients develop severe intestinal damage with diarrhea and bleeding, and eventually die of multiple organ failure, despite intensive organ support therapies [2].
Cyclooxygenase (COX) is known to exist in two isoforms, the constitutively expressed COX-1 and the inducible COX-2. The former is found in various tissues, including the small intestine [3], whereas the latter does not appear to be expressed, or occurs at markedly low levels in most tissues, but it is rapidly produced in response to growth factors and cytokines [4]. This tissue- specificity has presented the concept that COX-1 is critical for housekeeping action in the gastrointestinal mucosa, whereas COX-2 is responsible for inflammation [5]. COX-2 expression was found to be significantly increased in colorectal polyps and colorectal cancer, and COX-2 inhibitors reportedly inhibit tumor growth [6]. Accordingly, the usefulness of COX-2 inhibitors for chemoprevention in colorectal cancer has been carefully suggested [7]. It is well-established that the risk of colon cancer is relatively high with prolonged inflammation. Furthermore, the usefulness of COX-2 inhibitors could be even greater in inflammatory disease [7]. However, clinical studies are still lacking and additional investigations on stability are needed.
Celecoxib, a COX-2 selective non-steroidal anti-inflammatory drugs (NSAID), is used to control pain and inflammation in patients with osteoarthritis, rheumatoid arthritis, and ankylosing spondylitis, with numerous ongoing studies aimed to determine whether celecoxib is useful in those conditions [8]. However, during molecular studies in the laboratory, it has been apparent that celecoxib could also interact with other intracellular components in addition to its most notable target, COX-2. The discovery of these additional targets has generated considerable controversy, and the initial assumption that celecoxib reduces tumor growth primarily by COX-2 inhibition was disputed [8].
In the present study, we examined the histopathological changes in the gastrointestinal tract after celecoxib was administered to irradiated mice, and confirmed the expressional changes of COX-2 to determine the impact of COX-2 on the gastrointestinal tract when irradiation and celecoxib were co-administered.
Materials and Methods
Seven-week-old male BALB/c mice were purchased from Dooyeol Bio Laboratory (Chungbuk, Korea), and experiments were performed after one week of quarantine and acclimatization. All animal experiments were performed according to procedures approved by the Institutional Animal Care and Use Committee of the Korea Institute of Oriental Medicine (Approval No. 2020-032).
Mice were anesthetized using alfaxalone (Alfaxan®, Careside, Sungnam, Korea) and xylazine (Rompun®, Bayer Korea, Seoul, Korea), and restrained on a tray with the abdominal lesion extended outside the radiation field. Mice were exposed to subtotal body radiation using 6 MV high energy photon rays (ELEKTA, Stockholm, Sweden) at 3.8 Gy/min. Abdominal radiation at doses of 10 Gy was used to evaluate morphological changes in the jejunum. Sham irradiated mice were treated in an identical manner without radiation. Celecoxib was obtained as a powder from Pharmacia (St. Louis, MO, USA) and mixed in 0.5% methylcellulose and 0.025% Tween 20 in sterile water to obtain a concentration of 7 mg/mL. Mice were administered freshly prepared celecoxib via oral gavage at a dose of 25 mg/kg in 0.1 mL, daily for 4 days after irradiation exposure. The celecoxib dose was based on previously published results demonstrating its biologic effectiveness as a COX-2 inhibitor [9]. Control animals received the same volume of vehicle administered in the same manner, at identical time points.
24 male mice were divided into 4 groups (n = 6 per group) as follows: sham irradiated control mice, saline- treated 10 Gy-irradiated mice, celecoxib-treated mice, and celecoxib-treated 10 Gy-irradiated mice. Mice were sacrificed 3.5 days after radiation. The isolated small intestines were fixed in 10% neutral buffered formaldehyde and embedded in paraffin wax to prepare 4-µm-thick jejunal sections for hematoxylin-eosin staining. The regenerating crypts in the jejunal cross-sections were then counted by a previously described microcolony technique [10, 11]. The criteria for regenerating crypts is as follow: prominent nucleus, little cytoplasm, lying close together and appearing crowded [12]. To analyze morphological changes, all samples were sectioned and reoriented by successive slices to identify the sample with the longest villi. This sample was used as it yielded more homogenous results than standard techniques based merely on measuring the 10 longest villi from a single section of the sample. The lengths of the 10 longest villi, the number of crypts, and the crypt size from each sample were measured. Images of intestinal sections were obtained with a digital camera mounted on a Nikon Eclipse 80i microscope (Nikon, Tokyo, Japan). Quantification was performed using Image-Pro Plus image analyzing software (Media Cybernetics, Bethesda, MD, USA).
The immunohistochemistry protocol was based on a previous study [13]. Briefly, the 4-µm-thick jejunal sections were deparaffinized, and then endogenous peroxidase activity of the tissue was blocked using 0.3% (v/v) hydrogen peroxide for 20 min. Further, 5% (v/v) normal horse serum (Vector ABC Elite Kit; Vector Laboratories, Burlingame, CA, USA) was used for blocking non-specific reactions for 60 min. The sections were incubated at 4°C with a mouse anti-COX-2 antibody (18-7379, 1:200 dilution; Zymed Laboratories, South San Francisco, CA, USA) overnight. After extensive washing, the sections were incubated with biotinylated anti-mouse immunoglobulin G (Vector ABC Elite Kit; Vector Laboratories) for 60 min. The sections were then incubated for 60 min with an avidin–biotin peroxidase complex (Vector ABC Elite Kit; Vector Laboratories). After washing, diaminobenzidine substrate (DAB kit; Vector Laboratories) was used for the peroxidase reaction. For a suitable control, the primary antibody was omitted from a few test sections. The sections were counterstained with hematoxylin prior to mounting.
After treatment, the intestinal samples (n = 4 per group) were homogenized in a reaction buffer (150 mM NaCl, 20 mM Tris [pH 7.5], 1% NP40, 5 mM EDTA, 10 mM NaF, 1 mM Na3VO4, 1 mM DTT, and 1 × PIC). Extracts were assessed using the Bio-Rad protein assay kit (BIO-RAD, Hercules, CA, USA), with bovine serum albumin as the standard. Total protein equivalents for each sample (30 mg protein per lane) were separated in 10% SDS- polyacrylamide gels and electrophoretically transferred to an Immobilon-PSQtransfer membrane (Millipore, Bedford, MA, USA). The membrane was immediately placed in a blocking solution (5% nonfat milk) at room temperature for 60 min, followed by incubation with diluted primary antibodies for COX-2 (Rabbit; 1:1,000, Abcam, Cambridge, UK) and β-actin (Mouse; 1:1,000, Sigma-Aldrich, St. Louis, MO, USA) in TBS-T buffer (Tris-HCl buffer with 0.2% Tween 20, pH 7.5) at 4°C overnight. The next day, the samples were incubated with a secondary antibody, either polyclonal anti-rabbit antibody or monoclonal anti-mouse antibody (1:10,000; Invitrogen, Carlsbad, CA, USA) in TBS-T buffer at room temperature for 60 min. The horseradish-conjugated secondary antibody labeling was detected by enhanced chemiluminescence and exposure to radiographic film. The bands were quantified using Scion Image Beta 4.0.2 for Windows XP (Scion, Frederic, MD, USA).
Total RNA was isolated from intestinal samples using a Hybrid-R Kit (GeneAll Biotechnology, Seoul, Korea). RNA (1 μg) was subjected to reverse transcription with PrimeScript RT master mix, according to the manufacturer’s protocol (Takara, Tokyo, Japan). PCR was performed in triplicate using a CFX96TM Real-Time system (Bio-Rad) and qPCR SYBR Green master mix (m.biotech, Hanam, Korea). For each target gene, the relative expression levels were normalized to the average expression of β-actin (F-TCCTTCCTGGGTATGGAATCCTGT and R-CTCCTTCTGCATCCTGTCAGCAAT) using the ΔΔCT comparative method [14]. The following transcripts were measured using COX-2 primers (F-GAAGTCTTTG GTCTGGTGCCTG and R-GTCTGCTGGTTTGGAATAG TTGC).
Results
As shown in Fig. 1, at 3.5 days after radiation (10 Gy), the villus height was significantly lower in irradiated mice, revealing the injurious effects of radiation on the jejunum. Furthermore, the villus height was greatly decreased in the celecoxib-treated irradiation group than that in the irradiated group (Fig. 1A–E).
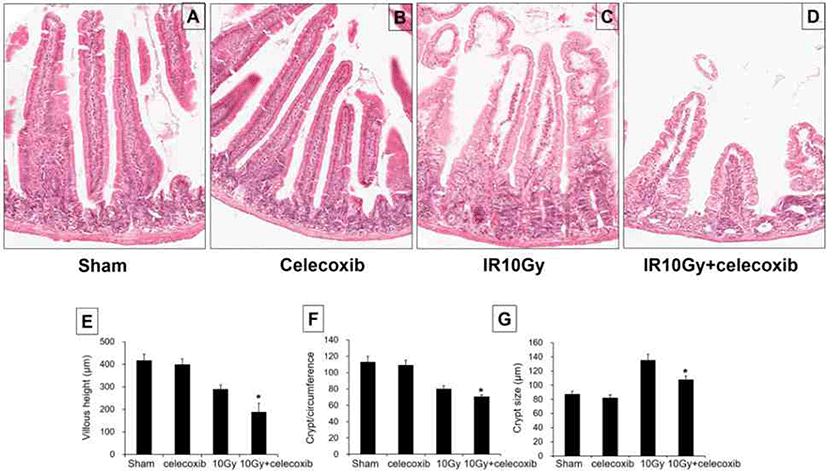
The morphology of the jejunal mucosa was altered 3.5 days after radiation exposure (10 Gy). The sham-irradiated mice and celecoxib-treated mice showed a large number of crypts at the base of villi. Irradiated mice displayed a markedly lower number of surviving crypts after 3.5 days. Celecoxib-treated irradiated mice showed a significantly decreased number of surviving crypts when compared with irradiated mice (Fig. 1A–D, and F).
The crypt size was greater in mice that received the irradiation, reflecting the intestinal response following injury, which eventually leads to repair. The crypt size was significantly lower in the celecoxib-treated irradiation group than that in the radiation group (Fig. 1A–D, and G).
The sham-irradiated control group showed minimal COX-2 expression in the intestine, with weak staining in some parts of the crypt and epithelial villi. Following irradiation, high levels of COX-2 were detected in the crypt lesion of the intestine. In celecoxib-treated irradiation mice, COX-2 expression was decreased in the crypt lesion (Fig. 2A–C, and D). Quantitative scoring of intestinal COX-2 expression is presented by Western blotting. Celecoxib administration to sham irradiated mice decreased the intestinal COX-2 expression levels. Whereas the COX-2 protein level was increased following irradiation. Administration of celecoxib after irradiation significantly decreased COX-2 protein levels when compared with the irradiation-only group (Fig. 2E and F). In the irradiated intestine, the mRNA expression level of COX-2 was markedly increased compared with the sham-irradiated group. Notably, celecoxib treatment in the irradiation group significantly reduced the mRNA expression of COX-2 when compared with that observed in irradiated mice; this effect was more pronounced than that following treatment with celecoxib alone (Fig. 2G).
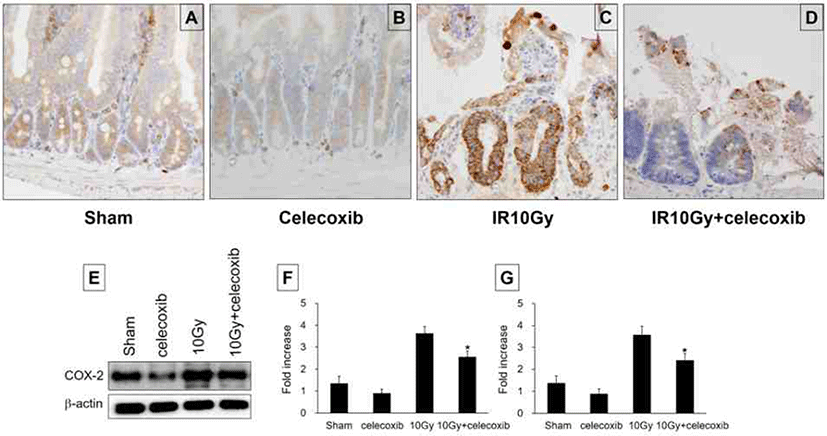
Discussion
In the present study, we evaluated the role of COX-2 in association with histopathological changes that occur following intestinal irradiation in mice. Previous studies have revealed that intestinal injury after irradiation can be evaluated based on the number of crypts, length of villi, and the size of crypts [10, 11, 13, 15]. The disappearance of intestinal crypts after radiation exposure occurs within 2 days, and a new intestinal shape appears following the recovery of surviving gastrointestinal stem cells 3 to 4 days after irradiation exposure [16]. Therefore, the present study investigated the intestinal injury after celecoxib treatment in irradiation group at 3.5 days after irradiation. The villous height, crypt number and crypt size were significantly decreased in celecoxib-treated irradiation group compared to that in the irradiation group. Thus, the degree of the intestinal injury in the celecoxib-treated irradiation group was more severe than that of the irradiation group. The mRNA and protein expressions of COX-2 increased after 10 Gy irradiation, whereas the expressions decreased in the celecoxib-treated irradiation group.
Gastrointestinal disorders induced by radiation therapy are one of the most important side effects observed. In patients with cancer, treatment and control of gastrointestinal disorders following radiation chemotherapy remains crucial [17]. A recent report suggests that anti-inflammation effect of antibiotic might alleviate the radiation-induced intestinal injury [18]. It has been also reported that celecoxib treatment with radiotherapy could increase the survival rate of tumor patient through inhibition of COX-2-derived prostaglandin E [19]. Furthermore, it has been shown that the activity of colitis was weakened and healing process was promoted following the administration of COX-2 inhibitors in an animal model [20]. These reports provide evidences that COX-2 expression may act toward aggravating inflammation. However, there are several contradictory studies on whether COX-2 expression exacerbates inflammatory processes or promotes mucosal regeneration. Arachidonic acid, a component of the cell membrane, undergoes metabolism by COX and results in the synthesis of prostaglandin. Prostaglandins, which are essential for maintaining homeostasis, are known to promote the healing of mucosal damage by inducing the proliferation of epithelial cells [21]. Additionally, prostaglandins trigger and regulate cytokines synthesis as a part of healing processes against the inflammation [22]. A large amount of prostaglandin has been detected in mucosal tissues and rectal dialysis fluid of patients with inflammatory bowel disease, with the prostaglandin amount and disease activity revealing a good correlation [23]. In intestinal epithelial cells, growth factors such as epidermal growth factor and transforming growth factor induce COX-2 expression, indicating that COX-2 will act as a defense factor in inflammation [24]. COX-2 reportedly plays a role in maintaining mucosal homeostasis and thus is involved in ulcer formation induced by NSAIDs in the stomach and small intestine [25]. In the dextran sulfate sodium-induced colitis model, selective COX-2 inhibitors exacerbate colon ulcers and inflammation [26]. Therefore, during inflammatory reaction, COX-2 is partially involved in mucosal protection, and selective COX-2 inhibitors may cause inflammatory disease exacerbation. However, it is still controversial about the exact roles of COX-2 in inflammation and related clinical prognosis, and it need to be further studied.
In this study, we observed that a clinically therapeutic dose of celecoxib significantly aggravated the severity of the radiation-induced intestinal injury. This indicates that the reduced COX-2 expression induced by celecoxib treatment could influence the risk of intestinal complications after pelvic or abdominal radiotherapy. Therefore, celecoxib treatment in combination with radiation therapy for cancer treatment needs to be careful, although the underlying mechanisms remain unclear.