Introduction
Colon cancer is the third most widespread cancer with high morbidity and mortality, and the fourth common reason of cancer deaths in the world [1]. In many publications, colon and rectal cancer have been researched collectively and the term, colorectal cancer (CRC), is used [1]. Diet would firmly affect CRC risk, and modifications in foods habits might lessen up to 70% of this cancer risk [2]. Published in 1997, the World Cancer Research Fund authoritative expert report states: “evidence shows that red meat probably increases risk and processed meat possibly increases risk of CRC” [3].
Since 2000, three meta-analyses suggested that total meat consumption has nothing to do with risk, but that red meat consumption is an important risk factor [4]. One of the leading hypotheses that have been studied experimentally is that heme-iron in red meat can trigger carcinogenesis because it arises cell proliferation in the colonic mucosa, through lipoperoxidation of feces [5]. Sesink insisted that the oxidative form (Fe3+) of heme, hemin represents that red meat intake has relevance to the risk of CRC [6]. Heme iron is thought to catalyze the endogenous formation of N-nitroso compounds in the colon, many of which are known carcinogens [7]. Therefore, excessive heme-iron can contribute to cause cancer in the colon.
Azoxymethane (AOM) and deodesyl sodium sulfate (DSS) are used to start the initiation of CRC by causing aberrant crypt foci (ACF). First reported in 1996, AOM/ DSS mouse model was proven as an outstanding model in faithfully mimicking of the pathological changes of “normal-ACF-adenoma-carcinoma”development as observed in human CRC, and hence was widely used in studies of colorectal carcinogenesis and chemopreventive intervention [8, 9]. ACF have emerged as a putative precursor to colorectal adenoma, and have been suggested to be a potentially useful biomarker for CRC [10]. Dietary heme iron (hemin) was found to cause similar colonic changes as meat-based diets in AOM-treated rats [11], and changes in gene expression linked to cancer and proliferation were detected in colon scrapings of mice after only 4 days of heme iron (hemin) administration [12]. In addition, Hemin and hemoglobin increase the number of AOM-induced ACF which are the previous stage colon cancer of rat [13].
Meanwhile, epidemiologic evidence suggests that vitamin C-rich foods play a protective role against development of cancer [14]. Plasma concentrations of ascorbic acid (AA) have been shown to be inversely associated with cancer risk [14]. The fact that AA is required to maintain full function of an array of enzymes indicates that optimizing intake would optimize metabolism as well as prevent cancer [15]. Although there are numerous studies about heme-iron effect on CRC model and influence of AA with iron, the interaction between AA and hemin in CRC is not evident. In this research, the protective effect of AA was investigated on pre-neoplastic lesions arose from AOM/DSS with hemin in mice.
Materials and Methods
AOM was obtained from Sigma-Aldrich (St. Louis, MO, USA). Dextran sodium sulfate (DSS) whose molecular weight is 36,000–50,000 was obtained from MP Biomedical (Irvine, CA, USA). Hemin (≥97.0%) which is powder forms was obtained from Sigma-Aldrich. L-ascorbic acid was obtained from Sigma-Aldrich.
Male ICR mice (4 weeks old) were obtained from Orientbio Laboratory Animal Seongnam, Korea) and housed in isolating polycarbonate cage (5 mice/cage). The temperature and relative humidity were maintained at 20 ± 2°C and 50 ± 20%. Light and dark cycles were at 12 h each and the intensity of illumination was maintained at 150–300 lux. The AIN-76A purified diet purchased from Central Animal Laboratory (Seoul, Korea) was given to the mice. During the experimental periods, diets and litter were all used after sterilization and the animal experiment was conducted in compliance with “Guide for care and use of Laboratory animals” of Chungbuk National University. During the experimental period, weekly body weight and water consumption were recorded. The experiment was approved by Chungbuk National University Institutional Animal Care and Use Committees with the approval number, CBNUA-81A-17-01.
After acclimation for 1 week, five-week old mice was divided into 3 groups (15 mice/group) including (1) carboxymethylcellulose (CMC): control group, (2) CMC + hemin group, (3) CMC + hemin + 1.0% AA dissolved in distilled water group. AIN-76A purified diet was fed to the three groups. Hemin (2 g/kg B.W.) mixed with 1.0% CMC was orally administrated to each group. Also, the mice were subcutaneously treated with AOM (10 mg/kg B.W.) three times at the 0, 1st, and 2nd weeks of the experimental period to induce the formation of pre-neoplastic lesion in colon. From the next day, drinking water containing 2.0% DSS was provided for 7 days and the total experimental period is 6 weeks (Fig. 1).
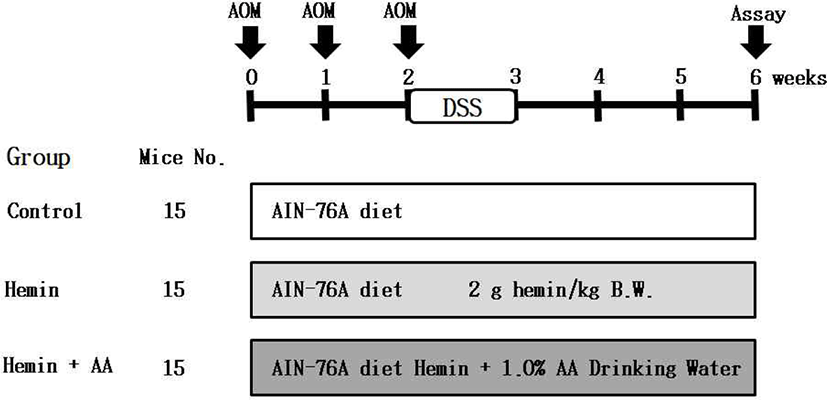
At 6 weeks after the experiment, all mice were sacrificed. After performing cervical dislocation, the colons were harvested and flushed with 0.9% NaCl solution and finally fixed in 10% neutral phosphate buffered formalin. The formalin-fixed colonic tissues were stained in 0.2% methylene blue solution for 30 sec. The numbers of ACF and aberrant crypts (ACs) were counted under a microscope (40–100 ×). ACF were identified via the following morphological characteristics: 1) enlarged and elevated crypts compared to normal mucosa and 2) increased pericryptal space and irregular lumen [16]. The total number of ACF was recorded as number/colon (Fig. 2).
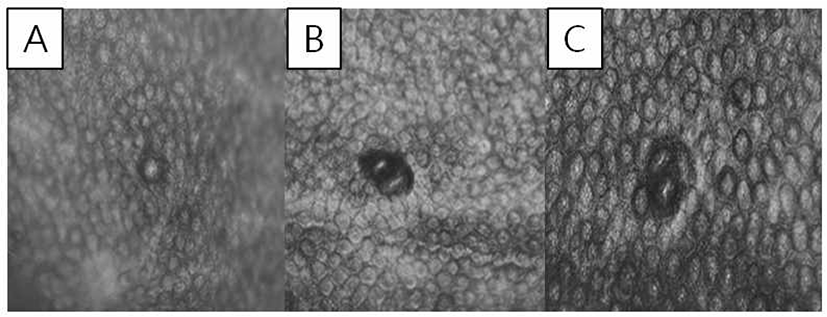
Feces sample was collected from 5 mice/one cage. One sample thus corresponded to the pooled feces from five mice. Feces were prepared by reconstituting freeze-dried feces by adding 1 mL of distilled water to 0.3 g of freeze-dried feces. Samples were incubated at 37°C for 1 h and were thoroughly mixed during the incubation time. They were then centrifuged for 15 min at 20,000 g, and supernatants were collected and stored at –20°C until use.
TBARS assay was conducted for determination of malondialdehyde of which is a by-product of lipid peroxidation in feces. At first, collected sample 100 μL, 8.1% sodium dodecyl sulfate (SDS) solution 100 μL, 20% acetic acid solution 200 μL were mixed together and 0.75% 2-thibarbituric acid solution 100 μL were added lastly and they were vortexed. Then, the incubation at 95°C was conducted for 1 hr and the centrifuge process was conducted at 4°C, 1,600 g for 10 min. At last, they were put in the 96-well plate and the optical density of the supernatant liquid was measured at 530 nm. Standards were used MDA concentrations at 0, 100, 0.625, 1.25, 2.5, 5, 10, 25, and 50 mM.
Results
The mice body weights of all experimental groups were increased with the passage of time. During the 6-week experimental period, hemin + AA group had lower body weights compared with the control groups and hemin group without a significance (Fig. 3).
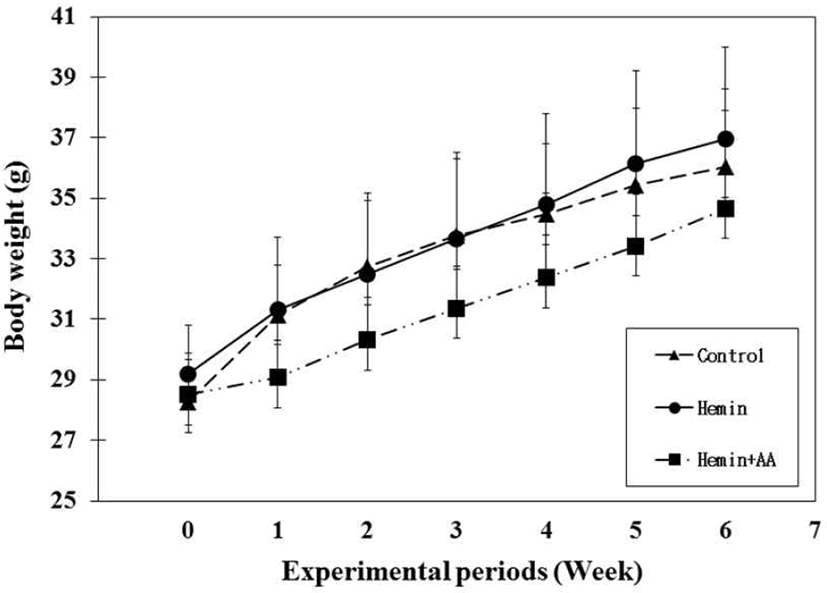
Total number of ACs in hemin group (31.2 AC/colon) were significantly increased compared to control group (14.9 AC/colon) (p<0.01). Total number of ACs in hemin + AA group (15.7 AC/colon) were significantly decreased compared to hemin group (p<0.01). But there was no a significant difference between control group and hemin + AA group (Fig. 4).
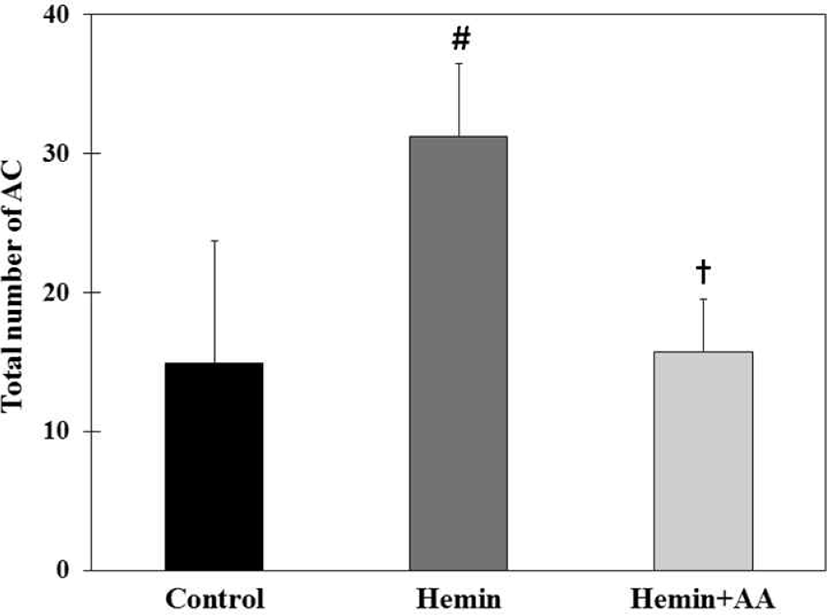
Total number of ACF in hemin group (23.7 ACF/colon) were significantly increased compared to control group (11.5 ACF/colon) (p<0.01). Total number of ACF in hemin + AA group (11.29 ACF/colon) decreased compared to hemin group (p<0.01). There was no significant difference in the number of ACs between control and hemin + AA group (Fig. 5).
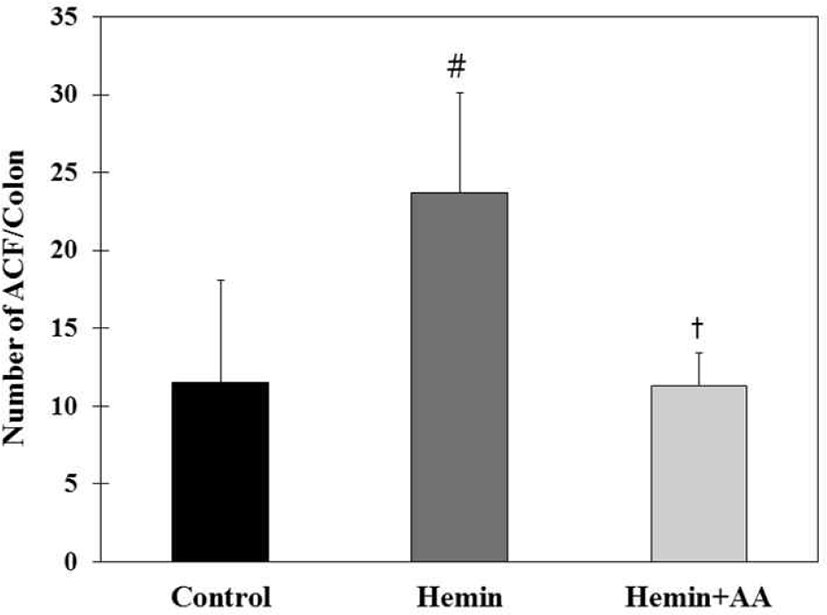
Lipid peroxidation was thus measured in fecal water by the TBARS assay. The TBARS values were the lowest in AOM/DSS control group among experimental groups (Fig. 6). Hemin treatment significantly increased the TBARS value compared with the control (p<0.01), but AA supplementation by drinking water significantly decreased the TBARS value compared with the hemin group (p< 0.01) (Fig. 6).
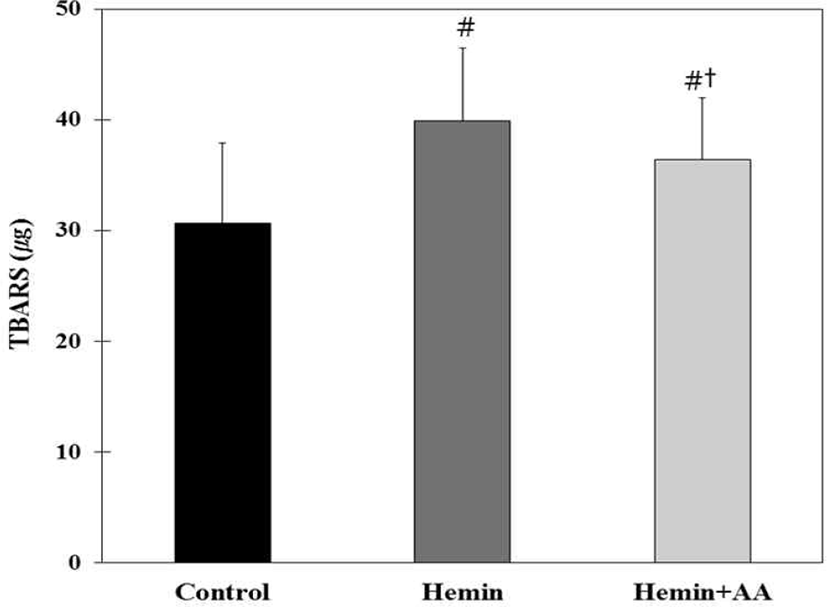
AOM treatment showed earliest dysplastic colon epithelium prior to tumor development. Hemin group showed a thicker epithelium and inflammatory infiltrates in colon mucosa. Hemin + AA group ameliorate the histopathological changes (Fig. 7).
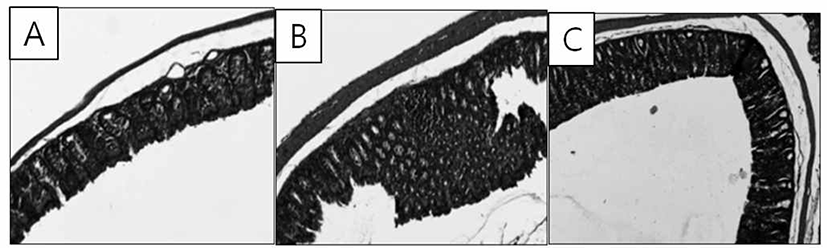
Discussion
The colon is the most prevalent site for neoplasms of the digestive tract, and CRC accounts for the second highest percentage of cancer mortality rate in males dwelling in developed countries [17]. It does not seem that colon cancer is a consequence of aging, but it is basically related to dietary habits. The consumption of red and processed meat increases the risk of CRC, and this assertion is discovered to be associated firmly with the consumption of dietary iron [18]. Dietary heme-iron significantly increased the colon malignancies in mice at the aberrant crypt stage. This result advocates the hypothesis that heme can explain the correlation between red meat consumption and colon cancer risk [19]. Certainly, this study showed that 1) heme as hemin increased the number of AOM/DSS-induced ACF in the colon of mice, 2) hemin effect was associated with lipid peroxidation in fecal water, 3) hemin effect was inhibited by the consumption of AA in drinking water. The effect of hemin and AA, as an antioxidant, is discussed below.
Hemin was used to analyze the effect of heme from red meat because meat hemoproteins are digested in the stomach and the small bowel, and provide free heme to the colon [20]. We investigated the influence of AA on the formation of colonic ACF induced by AOM/DSS in ICR mice. AA (1.0%) was administered via drinking water for 6 weeks. In the present study, hemin strikingly increased the number of AOM/DSS-induced ACF and ACs. Meanwhile, 1.0% AA treatment significantly decreased the number of total ACF and ACs compared to hemin group. ACF are putative pre-neoplastic lesions that have been detected in human colon cancer and experimental animals treated with chemical carcinogens. ACF can be identified microscopically on the surface of the whole mount colon mucosa after methylene blue staining. They are distinguished from normal crypts by their larger size, darker attaining, and increased perictyptal space [21]. In this study, the number of ACF was counted and the result shows that orally administered hemin surely negatively affected on the formation of colonic pre-neoplastic lesions in mice. On the other hand, the result in hemin + AA group, as it showed lesser numbers of ACF and ACs than those of hemin group, supports that AA was definitely effective on defensing the negative effect of hemin on colonic pre-neoplastic lesions in mice. These results suggest that AA supplementation via drinking water can inhibit the development of experimentally induced pre-neoplastic lesions. The role of AA is likely to involve several functions. It acts as an electron donor and as a reducing agent which allows it to have a cytotoxic effect. The pharmacologic doses of AA decrease the proliferation of colon cancer cells and induce their apoptosis and necrosis [22].
One attribute of red meat that has been studied extensively to determine its potential contribution to colon cancer development is iron. Most studies evaluate iron impacts using hemoglobin or other heme compounds as a surrogate for the heme-containing proteins in meat. Heme catalyzes the formation of lipid oxidation end-products, which may explain the promoting effects of red and processed meats on CRC [23]. In the present study, lipid peroxidation in feces was evaluated by the TBARS assay. TBARS results from lipid peroxidation of polyunsaturated fatty acids and is a marker for oxidative stress [23]. In this study, the AOM/DSS control group showed the lowest level of TBARS but orally-administration of hemin increased the TBARS value in the fecal water. Fecal water-soluble components derived from hemin fed rats led to higher lysis of erythrocytes, suggesting hemin promoted a cytotoxic environment [24]. The resulting epithelial surface injury induced by hemin or its metabolites led to an increase in cell proliferation and a reduction in apoptosis to maintain epithelial barrier function [24]. In this study, there is a tendency to decrease the formation of lipid oxidation end-products when AA was supplied via drinking water, in combination with hemin treatment. Therefore, although the level of TBARS in hemin + AA group was not as low as that of control group, it is possible to say that AA suppressed the hemin-induced cytolytic activity of fecal water.
In conclusion, when hemin was administered to mice, there is a tendency to increase the formation of ACs, ACF and lipid oxidation end-products. However, the treatment with AA via drinking water likely decreased the increased formation of pre-neoplastic lesions in mice. From these results, it is possible to conclude that AA has a defense effect on meat-promoted colon cancer in human and animals. Further research might be required to prove the detailed mechanisms.