Introduction
Sepsis in one of the main causes of acute respiratory distress syndrome (ARDS), in which lungs are injured by circulating inflammatory mediators [1–3] that result in severely impaired gas exchange which usually requires invasive mechanical ventilation (MV). MV is one of the best treatments in patients with sepsis related to ARDS to achieve adequate gas exchange for patients with respiratory difficulties [4–7]. Despite the life-saving potential of MV, clinicians are aware of its several potential drawbacks and complications. ARDS can result in poor lung compliance with heterogeneous distribution, making less-affected lung areas more vulnerable to ventilator-induced lung injury (VILI). Moreover, MV itself can induce lung injury, bronchopulmonary dysplasia, and multiple organ failure [3, 8]. Characterization of potential interaction between sepsis and VILI requires appropriate animal models that can recapitulate the proinflammatory cascade seen in human and allow for testing of priming septic insult on the development of subsequent VILI [9–11]. However, studies that evaluate lung injury and function based on severity of sepsis, tidal volume of ventilation, and ventilation after sepsis induction are lacking. Thus, the objective of this study was to use a mouse cecal ligation and puncture (CLP) model of sepsis with subsequent injurious ventilation to determine if sepsis could affect the development of VILI. For that, we evaluated lung compliance and performed bronchoalveolar lavage (BAL) analysis in mice with CLP alone, MV alone through small animal ventilator, and MV after CLP or sham.
Materials and Methods
Adult (10–12 weeks old) healthy male and female C57BL/ 6 mice (weight, 22–30 g) were used. All mice were anesthetized by intraperitoneal injection of ketamine (100 mg/kg)/xylazine (10 mg/kg). These mice were maintained in pathogen limited conditions at room temperature. They were provided ad libitum access to water and commercial food. All animal procedures were carried out in accordance with the guidelines of the animal care and use committee of Chonnam National University (CNU-IACUC-YB-2014- 25).
To evaluate lung injury caused by sepsis only, three different degrees of sepsis were induced in mice. First, survival time was determined. Mice were assigned to three groups based on the severity of sepsis (1, mild sepsis; 2, moderate sepsis; and 3, severe sepsis) as described previously [12]. In our experiments, the cecum was ligated at different lengths below the ileocecal valve to determine severity. Animals were first anesthetized with Zoletil® (Tiletamine HCl and Zolazepam HCl, Virbac) by intraperitoneal injection. The abdominal area of each mouse was shaved and disinfected. After midline laparotomy, the cecum was exposed and specified percentage of its total length was ligated with 3-0 sterile Nylon. Ligation of 20% (n = 8), 50% (n = 6), and 70% (n = 7) induce mild, moderate, and severe sepsis, respectively (Fig. 1). The cecum was also punctured with sterile 18G needle and then gently compressed to extrude a small amount of cecum content. Next, the cecum was returned to the abdominal cavity. The peritoneum was sutured and 1 mL of sterile phosphate-buffered saline (PBS) was injected intraperitoneally for fluid resuscitation. All surgical procedures were done with sterile materials. Sham (n = 6) procedure consisted of laparotomy without cecum manipulation or perforation. After investigating survival time depending on sepsis severity, lung injury was measured at 24 h of sepsis for mild (n = 7), moderate (n = 12), and severe (n = 6) CLP model (Fig. 2a).
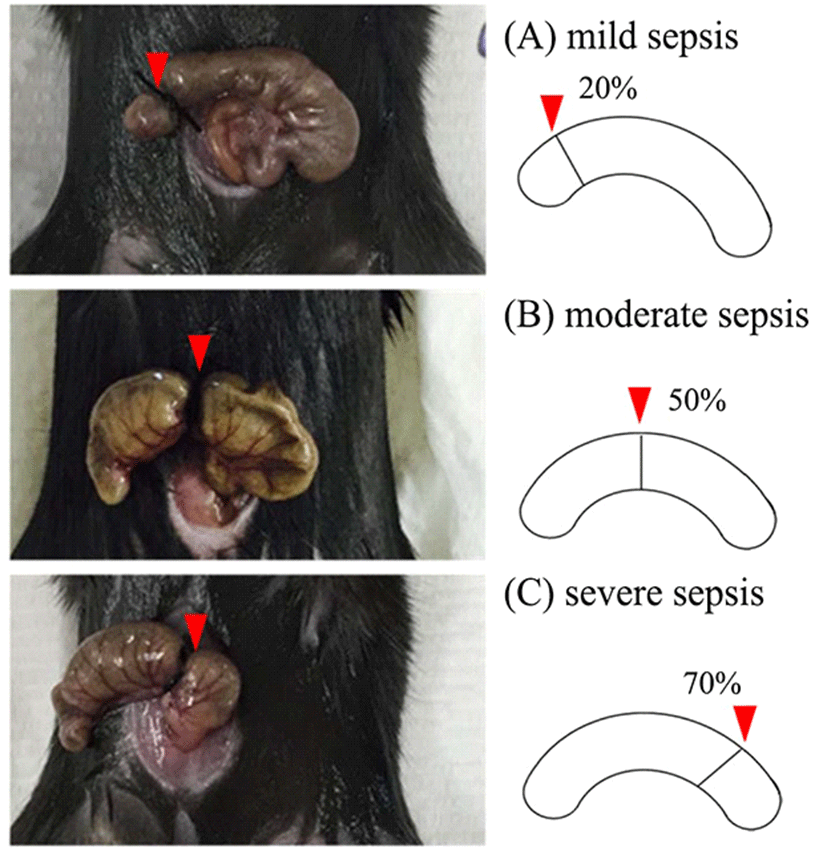
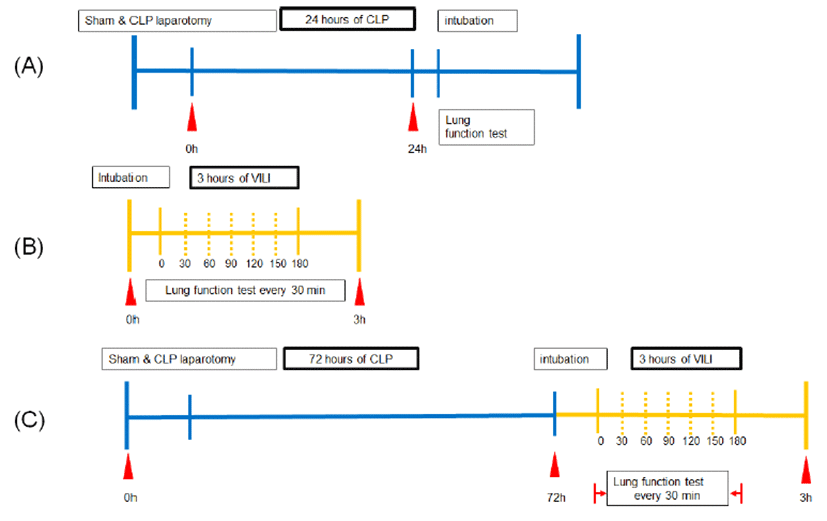
To evaluate lung injury caused by MV with or without sepsis, various tidal volumes (7 mL/kg, 10 mL/kg, or 24 mL/kg) were maintained for 4 h with or without septic insult and lung injury was then evaluated. In this study, mice were subdivided into three groups: low tidal volume (LTV, n = 7); normal tidal volume (NTV, n = 8); and high tidal volume (n = 8). Mice were mechanically ventilated using a small animal ventilator (FlexiVent, SCIREQ, Montreal, Quebec, Canada). The trachea was exposed under sterile condition, cannulated with a 22-G angiocatheter, and sutured. Animals were then connected to a SCIREQ Flexivent that allowed simultaneous ventilation and data capture. Immedifate closed chest ventilation was performed with the following parameters: respiratory rate, 150 /min; positive end-expiratory pressure (PEEP), 3 cm H2O; tidal volume, 7 mL/kg (LTV), 10 mL/kg (NTV), or 24 mL/kg (HTV) during 3-hour experiment period. For VILI, lung function test was measured every 30 min during the experiment (Fig. 2b). To investigate lung injury after sepsis, mild CLP for 72 h (n = 5) or sham (n = 5) was induced and then 30 min of HTV MV was applied before lung injury was investigated (Fig. 2c).
“Primewave-8” perturbation using a small animal ventilator (FlexiVent, SCIREQ, Montreal, Quebec, Canada) was performed until three acceptable measurements (coefficient of determination > 0.95) were recorded for each individual subject, of which an average was calculated [13–15]. Before taking measurements, a “Deep inflation” was run to recruit closed lung areas and standardize lung volume. Snapshot perturbation was imposed to measure compliance tissue damping (compliance) (G) and tissue resistance (H) of the respiratory system (airways, lung, and chest wall).
To recover intrapulmonary inflammatory cells, 1 mL of sterile PBS was instilled into lungs through the trachea and BAL was aspirated back. This procedure was repeated three times. BAL was centrifuged at 1,200×g for 10 min at 4℃ and total protein concentration was measured using a BCA Protein Assay Kit (Pierce, Thermo Fisher Scientific Grand Island, NY, USA) following the manufacturer’s experimental protocols. The assay was performed at room temperature and optical absorbance was measured at 570 nm using a microplate reader. Cells were stained with Trypan blue solution (Sigma-Aldrich, St. Louis, MO, USA) and counted with a hemocytometer.
All statistical calculations were performed using SPSS version 21.0 (SPSS Inc., Chicago, IL, USA). Shapiro-Wilk test was applied for normality test. One-way analysis of variance (ANOVA) was used for multi-group comparisons and Bonferroni post hoc test (parametric) following oneway ANOVA or Kruskal-Wallis (non-parametric) test was applied. Repeated two-way ANOVA and Student’s t-test (parametric) or Mann-Whitney U test (non-parametric) was also applied. Statistical significance was considered when p-value was less than 0.05.
Results
On average, mild, moderate, and severe sepsis groups survived 72 h, 21 h, and 13 h, respectively. Higher severity of CLP-induced sepsis reduced the survival time (Fig. 3). Lung compliance was significantly decreased with increasing severity of sepsis. In addition, the severe sepsis group had significantly higher concentration of BAL protein compared to the moderate sepsis group (Fig. 4). However, there was no significant difference in total cell counts in BAL among groups.
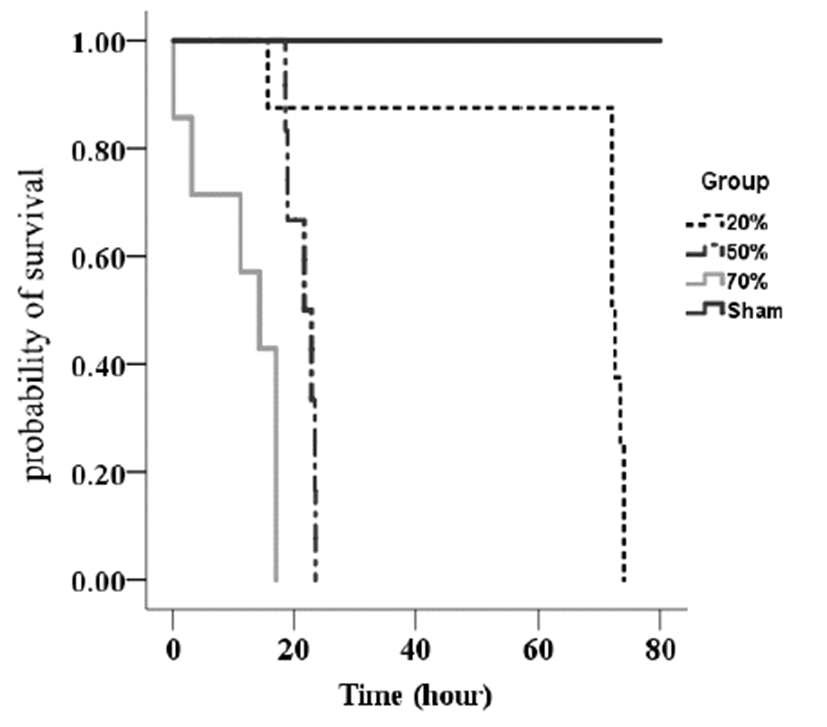
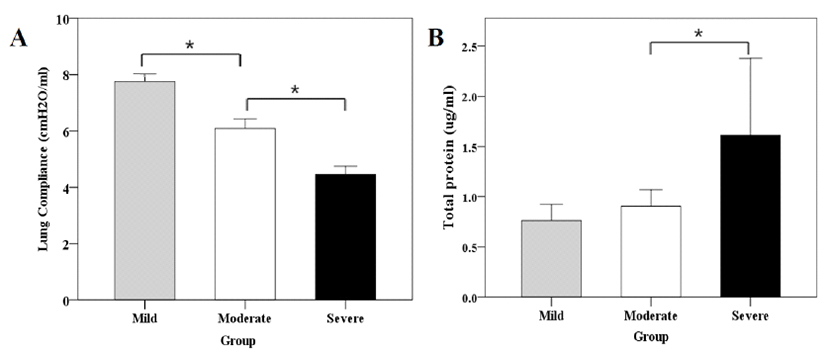
For 180 min of MV, lung compliance was significantly (p = 0.02) decreased in the HTV group. The HTV group also had significantly higher number of total cells compared to the LTV or the NTV group (Fig. 5). However, no significant difference in BAL concentration was found among the three groups.
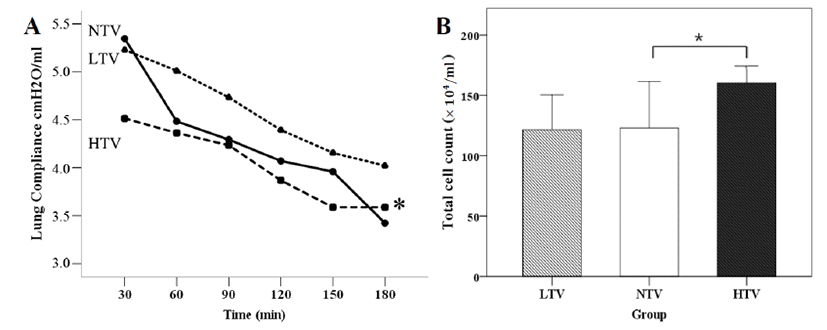
When 3 h of MV was applied to mice with 72 h of mild CLP induction, there was a significant difference in lung compliance between the sham group and the CLP group at 180 min after MV. Protein concentrations of BAL were consistently higher in all mice of the CLP group than those in the sham group (Fig. 6). Total cell counts were slightly decreased in the sham group compared to those in the CLP group, although the difference was not statistically significant.
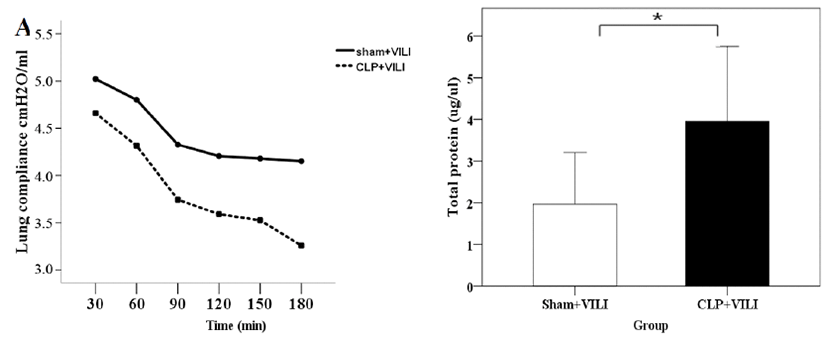
Discussion
The focus of this study was to observe acceleration of lung injury caused by ventilators in a mouse sepsis model. Lung compliance measurements, and BAL analysis (protein concentration, total cell count) were used as parameters for assessing the degree of lung injury. In order to determine tidal volume capable of inducing lung injury in mice, a VILI model using a small animal ventilator was designed.
In order to investigate lung injury upon septic insult, lung injury was investigated in mice under various conditions. When we determined the degree of severity of sepsis, ventilation could not be carried out due to short time of survival. Lung compliance could not be accurately measured due to severe systemic inflammation with anesthetics difficulties in moderate sepsis and severe sepsis groups. As a result, CLP 20% group was chosen as the default CLP model for VILI over the CLP model. Severe sepsis with HTV was not a proper animal model since mice did not survive before measuring lung function whereas mild sepsis for 72 h with 3 h of additional ventilation was an appropriate animal model for this study. The mild sepsis group survived an average of 72 h. The moderate sepsis group survived an average of 21 h while the severe group had a survival time of 13 h. Therefore, higher severity of CLP-induced sepsis reduced the survival time of mice after CLP induced sepsis. In addition, we identified the specific tidal volume that could induce lung injury in the VILI model. Finally, acceleration of VILI was evaluated and compared between the sham group and the CLP group in this study.
In sepsis, bacteria may spread through systemic circulation, leading to systemic inflammatory reactions. Reactive oxygen species (ROS) produced by neutrophils particularly plays a critical role in inflammatory reaction and tissue injury. In worst case scenarios, ROS can cause multiple organ failure and death in patients with acute lung injury or ARDS [16, 17]. CLP model was chosen as the experimental model of sepsis in this study. Compared to LPS model in which systemic response in animal models differs greatly from humans depending on the dose and route of LPS injection, the CLP model resembles progression and characteristics of human sepsis more closely [3, 18].
We determined lung compliance of mice with CLP-induced sepsis at 24 h after using FlexiVent (SCIREQ) system. Similar study has been done previously [19]. However, direct lung function was not measured. FlexiVent system is considered as a gold standard for measuring lung function. Lung compliance was significantly decreased in the moderate sepsis group compared to that in the mild sepsis group in the present study. It was significantly reduced in the severe sepsis group compared to that in the moderate sepsis group. Therefore, lung compliance was decreased when the severity of CLP-induced sepsis was increased. Protein concentrations of BAL were consistently higher in all mice subjected to CLP than those in the control group. The severe sepsis group had significantly higher total BAL protein compared to the mild or moderate sepsis group, indicating more lung injury in severe sepsis.
A ventilator is an essential medical device designed to artificially control respiration and achieve gas exchange within lungs of patients who are unable to breathe or cannot breathe sufficiently [20, 21]. However, using ventilators for extended periods of time may also cause lung injury and adverse effects [7]. VILI is an acute lung injury caused by high tidal volume and low end-expiratory lung volume during MV. If the tidal volume is set too high during ventilation, it may induce hyperinflation and stretch injury of the alveoli. These inflammatory agents may not only induce injury to lung tissues, but also permeate capillary membranes and spread inflammatory agents to other parts of the body through systemic circulation. Thus, MV itself can directly induce further lung injury while accumulated inflammatory agents and cytokines can indirectly cause secondary injury and necrosis of the vascular endothelium. Several studies have confirmed that death in patients with ARDS is more likely to be caused by multiple organ dysfunction than respiratory failure and that death rates are higher in ARDS patients with accompanied organ failure [8, 20]. Indeed, there was a significant (p = 0.02) difference in lung compliance between the NTV group and the HTV group in the present study. Total cell counts in the HTV group were significantly increased compared to those in the LTV or the NTV group.
Finally, lung compliance of the sham group was significantly lower than that of the CLP group at 3 hours after MV. Protein concentrations of BAL were consistently higher in all mice subjected to CLP than those in the sham group. Total cell counts were slightly decreased in the sham group compared to those in the CLP group.
Eventually, both CLP and VILI groups showed significant decrease in lung compliance compared to the sham group. They also showed significant increase in total cell count upon BAL. These results suggest that VILI is closely associated with cellular infiltration and that ventilation can accelerate lung injury in sepsis models. Further studies are needed to determine the mechanisms involved in such increase.