Introduction
Intercellular junctions consist of gap junctions, adhering junctions, and tight junctions (TJs). Gap junctions chemically and electrically link adjacent cells, adhering junctions form the cortical cytoskeleton under the plasma membrane and help to regulate molecular movement, and TJs comprise the intercellular barrier [1, 2]. Collectively, these junctions modulate intracellular and intercellular signaling and transport [3]. TJs perform a number of roles, including acting as barriers against the extracellular environment, regulating cellular permeability through cell-to-cell gaps, and serving as intermediates/transducers in cell signaling cascades [4, 5].
TJ proteins are commonly classified into three types: transmembrane, cytoskeletal, and cytoplamic plaque proteins [6]. Claudins (CLDNs), occludin (OCLN), and junction adhesion molecule A (JAM-A) are transmembrane proteins, whereas zona occludens-1 (ZO-1) is a cytoplasmic plaque protein [7]. Transmembrane proteins regulate paracellular permeability for various factors such as water, solutes, and immune cells and form a second barrier that controls cell polarity by limiting exchange of membrane lipids [8].
CLDNs are integral transmembrane proteins that comprise a family of at least 27 members [9]. CLDNs have been detected in numerous organs, including the duodenum, ileum, colon, kidneys, liver, lungs, and skin [10, 11]. CLDN internalization is known to be caused by toxins, including Escherichia coli cytotoxic necrotizing factor-1, Helicobacoer pylori-associated factors, and Clostridium perfringens enterotoxin [12]. Inflammatory cytokines have been found to reduce CLDN expression and subsequently increase paracellular permeability [13]. Abnormal expression of CLDNs has been observed in the case of cancer or tumors and may be associated with cancer development and metastasis. OCLN is a major transmembrane protein with extracellular loops containing unique charged structures that may enable cells to easily attach to each other [14]. OCLN acts as a paracellular barrier, maintains cell surface polarity through interaction with ZO-1, and regulates the permeability of endothelial cells [15, 16]. Staphylococcus aureus α-toxin [17], Botulinum toxin [18], Clostridium difficile toxin [19], and enteropathogenic Escherichia coli toxin are known to affect OCLN expression and cause disease [20]. In addition, increased expression of OCLN is associated with Alzheimer's disease and vascular dementia [21]. Dysregulated OCLN expression is also related to tumorigenic and metastatic properties of cancer cells [22]. Expression of OCLN protein has been reported in many organs, including the brain, testis, epididymis, kidney, liver, lung, stomach, duodenum, ileum, colon, skin, and blood vessels [23, 24]. JAM-A is an integral transmembrane protein belonging to the immunoglobulin superfamily and is closely associated with immune responses. This protein is expressed on the surfaces of leukocytes, platelets, and erythrocytes [25]. JAM-A has many functions, including intercellular junction assembly, paracellular barrier formation, promotion of leukocyte migration, platelet activation, angiogenesis, and reovirus binding [26]. Expression of this protein has been detected in numerous organs, including the duodenum, ileum, colon, kidneys, lungs, liver, brain, muscle, trachea, and pancreas [27, 28]. Abnormal JAM-A expression causes intestinal diseases such as colonic inflammation, increased mucosal permeability, lymphoid follicle hyperplasia, and irregular epithelial cell proliferation [29]. Moreover, cancer development and metastasis [30], viremia [31], and platelet disorders [32] are known to be associated with JAM-A.
ZO-1 is a cytoplasmic plaque protein containing PDZ domains, which adhere to integral membrane proteins and connect the proteins to each other [33]. ZO-1 has three alternative splicing domains: α, β, and γ [34]. ZO-1 protein interacts directly with transmembrane factors, including CLDNs, OCLN, and JAM-A, and is known to organize and regulate the structure of TJs [2]. Toxins and pathogens, including Clostridium difficile toxin A [35] and Entamoeba hystolytica [36], are known to alter expression of ZO-1 and consequently epithelial permeability. Many studies have demonstrated that this protein is associated with tumor development and metastasis [37], gastrointestinal disease [38], and embryonic development [39]. ZO-1 is expressed in numerous organs, including the lungs, brain, muscle, liver, kidneys, duodenum, ileum, and colon [40].
TJ proteins are physiologically important and have been linked to many diseases. Accordingly, numerous investigations have been conducted to examine the expression and regulation of TJ proteins in animals and humans. However, few of these studies have evaluated dogs, and none have compared mRNA and protein expression in canine organs.
Therefore, we measured the expression and localization of CLDNs (CLDN1, CLDN2, CLDN4, and CLDN5), OCLN, JAM-A, and ZO-1 in dogs. The uterine mRNA and protein expression of these factors was measured using reverse transcription (RT) PCR, quantitative real-time PCR (qPCR), and Western blot analysis. Localization of these molecules in canine organs was determined by immunohistochemistry.
Materials and Methods
Three 3-year-old intact female beagle dogs were sacrificed for our experiments. The dogs were fed a commercial diet (Natural balance, Korea) and tap water in stainless steel cages in a controlled environment maintained on a 12-hour light/dark cycle (temperature 23 ± 2°C, relative humidity 50 ± 10%, ventilation 17 ± 1 times/min). The dogs were euthanized by KCl, and a midline incision was made to collect the samples from the uterus. Institutional Animal Care and Use Committee (IACUC) of Chungbuk National University approved all experimental procedures.
The organs were put into Trizol reagent (Invitrogen, U.S.A.) and homogenized by ULTRA-TURRAX (IKA-Works, Germany). RNA was extracted from homogenate according to the manufacturer’s instructions. Then, 1 μg of RNA was reverse transcribed by first-strand moloney murine leukemia virus (MMLV) reverse transcriptase (iNtRON Bio, South Korea) and a random 9mer primer (TaKaRa Bio Inc., Japan) to synthesize complementary DNA (cDNA).
For reverse transcription (RT)-PCR and quantitative real-time PCR, β-actin, a housekeeping control gene, was used as an endogenous reference for normalization and measurement of relative gene expression levels.
In the RT-PCR procedure, genes were amplified in a 20 μL PCR reaction containing 1 U of i-StarTaqTM DNA polymerase (iNtRON Bio, Korea), 1.5 mM MgCl2, 2 mM dNTP, and 20 pmoL of primers. PCR reaction parameters were 30 cycles of denaturation at 95°C for 30 sec, annealing at 58°C for 30 sec, and extension at 72°C for 30 sec. Then, PCR products (10 μL) were separated on a 2.3% agarose gel and stained with ethidium bromide. Gel photographs taken under UV illumination were scanned using a Gel Doc EQ system (Bio-Rad Laboratories, U.S.A.).
In the qPCR procedure, 1 μL of each cDNA was assayed by SYBR PCR (TaKaRa Bio Inc., Japan) or the TaqMan (Applied Biosystem, U.S.A.) probe method quantitative real-time PCR (qPCR) according to the manufacturer’s instructions. qPCR was accomplished under the following conditions: 40 cycles of each denaturation at 95°C for 15 sec, annealing at 58°C for 15 sec, and extension at 72°C for 30 sec. Data for each sample were analyzed by comparing CT values at a constant fluorescence intensity. Transcription levels were inversely related to the observed CT. For every 2-fold dilution of the transcript, CT was expected to increase by one increment. Relative expression (R) was calculated using the equation: R=2–[ΔCT sample-ΔCT control].
The organs were put into Pro-prep (InTron Inc., South Korea). SDS-acrylamide gel (5~12.5%) was transferred to a PVDF membrane (PerkinElmer, U.S.A.). The membrane was then incubated overnight with primary antibodies against the following factors: CLDN1, CLDN2, CLDN5 (1:1000, mouse monoclonal, Invitrogen Co., U.S.A.), CLDN4 (1:500, rabbit polyclonal, Invitrogen CO., U.S.A), OCLN, ZO-1 (1:1000, rabbit polyclonal, Invitrogen Co., U.S.A.), JAM-A, and β-actin (1:1000, rabbit polyclonal, Santa Cruz Biotechnology, U.S.A.) diluted in BSA overnight at 4°C. Secondary antibody conjugated with horseradish peroxidase (mouse polyclonal, BIOSS, U.S.A. or rabbit polyclonal, Santa Cruz Biotechnology, U.S.A.) was diluted 3000-fold in 2.5% skim milk dissolved in TBS-T for 2 hours at room temperature. The blots were developed by incubation with ECL chemiluminescence reagent (Santa Cruz Biotechnology) and exposed to BiomaxTM Light film (Kodak, Rochester, NY, U.S.A.) for 1 to 5 min. Signal specificity was confirmed by blotting without primary antibody, and bands were normalized to β-actin. Signal intensity for each band was measured using NIH ImageJ software (National Institutes of Health, Bethesda, Maryland, U.S.A.).
Organ-specific localization of TJ molecules was investigated by immunohistochemistry. Embedded block was sectioned at 3.5 μm. Each slide was boiled in antigen retrieval solution (citrate buffer, pH 8.0) for 20 min. The slide was washed with TBS-T for 5 min. To block endogenous peroxidase, the slide was placed in 3% hydrogen peroxide for 30 min at room temperature. To stop the non-specific reaction, sections were incubated with 10% goat serum (Vecter Laboratories, Burlingame, CA, U.S.A.) in PBS for 1 hour at room temperature. The slides were incubated with primary antibodies, which were used in Western blotting overnight at room temperature in a moist chamber. Each primary antibody, CLDN1, CLDN2, CLDN4, CLDN5, OCLN, JAM-A, and ZO-1, was diluted 200-fold in BSA. Biotinylated secondary antibodies (1:400, mouse or rabbit IgG, Vector Laboratories, Inc.) were added and incubated at 37°C for 2 hours. Slides were again washed the same as above after incubation with primary antibody. ABC Elite (Vector Laboratories, U.S.A.) was added to slides and incubated at 37°C for 2 hours. Diaminobenzide (DAB; Sigma-Aldrich, U.S.A.) was used as a chromogen. Counter staining was achieved by Harris hematoxylin (Sigma-Aldrich, U.S.A.).
Results
Uterine mRNA expression of TJs (CLDN1, CLDN2, CLDN4, CLDN5, JAM-A, ZO-1, and OCLN) was analyzed by RT-PCR and qPCR (Fig. 1). β-actin was used as an internal control. The results show that expression patterns of TJs varied among genes. The upper band was originated by RT-PCR, and quantification was performed using qPCR data. CLDN1, CLDN4, JAM-A, ZO-1, and OCLN were expressed in the uterus, with OCLN showing the highest expression.
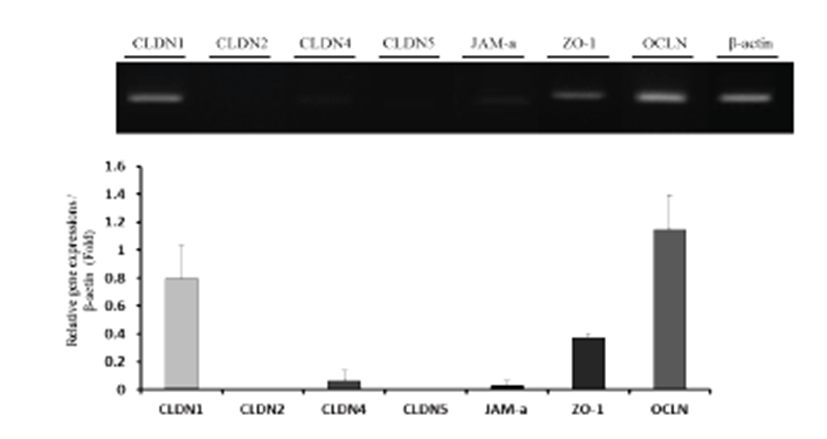
Uterine protein expression of TJs (CLDN1, CLDN2, CLDN4, CLDN5, JAM-A, ZO-1, and OCLN) was analyzed by Western blotting (Fig. 2). β-actin was used as an internal control. Western blotting showed similar gene expression (CLDN1, CLDN4, JAM-A, ZO-1, and OCLN). In contrast with mRNA expression, JAM-A protein showed the highest expression level in the uterus.
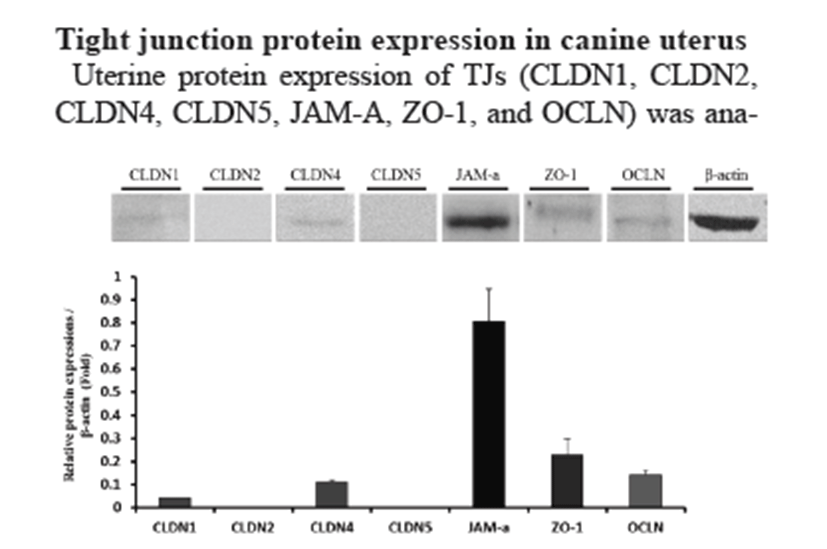
To examine the exact expression site of uterine TJs and determine their functions, we conducted immunohistochemistry of the canine uterus using antibodies specific for CLDN1, CLDN2, CLDN4, CLDN5, JAM-A, ZO-1, and OCLN (Fig. 3). Uterine tissues were composed of two compartments, the endometrium and myometrium. The endometrium included glands, epithelium, and stroma. CLDN1, CLDN4, and OCLN were localized to the uterine stroma, whereas JAM-A was specifically expressed in the endometrial gland epithelium and uterine epithelium and ZO-1 was expressed in the myometrium.
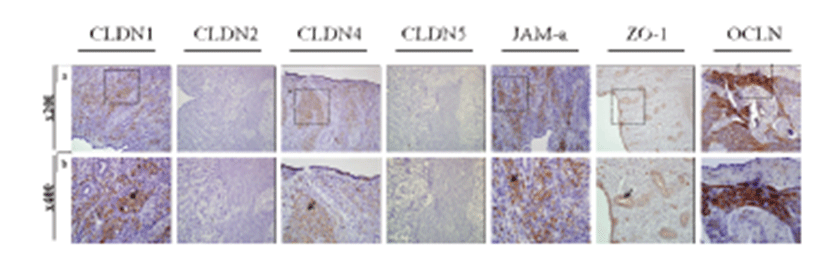
Discussion
Domestic animals are characterized as being non-menstruating and have conceptuses with a non-invasive type of implantation. These two distinguishing characteristics indicate the unique importance of elasticity of function in the uterine epithelia. The epithelia must adapt to establish an appropriate microenvironment based on the physiological status of the animal. TJs are the most apical structure of intercellular junctions and perform important roles such as intercellular barrier formation and regulation of cellular permeability and polarity. The structures of these proteins are similar, and the various physiological functions of the factors are facilitated by protein-protein interactions. Numerous studies on the expression, regulation, and cooperation of TJ molecules have shown that they modulate solute and water leakage (paracellular transportation). These micro-environmental modulations could affect uterine functions. In previous studies, various CLDNs were shown to be modulated in the uterus [41, 42]. Although various investigations have focused on these molecules in humans and lab animals, none have compared TJ factors in the canine uterus.
In the current study, we examined the organ-specific expression and localization of transmembrane proteins (CLDNs, OCLN, and JAM-A) and a cytoplasmic plaque protein (ZO-1) in the canine uterus. In a previous study, organ-specific variations in trans electrical resistance (TER) and paracellular ionic selectivity among epithelia were determined by the differential expression and distribution of CLDNs [43]. Similar to CLDNs, expression and distribution patterns of OCLN, JAM-A, and ZO-1 were different from those in other animals and humans [24, 27, 28, 39]. In this study, CLDN1 and CLDN4 were detected in the endometrial stroma. TJs of the uteri regulate the paracellular pathway between the uterine lumen and gland. Many studies have reported a correlation between TJ distribution and uterine physiology. Mendoza-Rodríguez and González-Mariscal determined that TJ distribution in the rat uterine epithelium changes during the estrous cycle, which suggests that the junctions help maintain an environment necessary for successful fertilization [44]. Megan and Christopher also observed the distribution patterns of TJs in rat uterine epithelial cells during early pregnancy and found that TJs influence implantation by controlling the uterine luminal environment [45].
In conclusion, our results show that canine TJ proteins, including CLDNs, OCLN, JAM-A, and ZO-1, were expressed in the canine uterus. Our data suggest that these proteins may perform unique physiological roles in the uterus. Therefore, these findings may serve as a basis for further studies on TJ proteins and their roles in the physiological or pathological condition of the canine uterus.