Introduction
Poria cocos is a well-known traditional Chinese traditional medicine (TCM) that grows around the roots of pine trees in China, Korea, Japan, and North America [1]. Poria cocos has been used in Asian countries to treat insomnia as either a single herb or part of an herbal formula [2]. Earlier studies have shown that the main ingredients of this herbal medicine are triterpenoids. Pachymic acid (PA), a lanostane-type triterpenoid, possesses anti-emetic, anti-inflammatory, and anti-cancer properties [3-8]. Recently, we reported that PA also enhances pentobarbital-induced sleeping behaviors [9].
Insomnia is a highly prevalent condition defined as perceived inadequate sleep, with patients typically presenting difficulty falling asleep, difficulty maintaining sleep, or poor quality sleep [10]. Due to the widespread nature of this condition, more effective treatments are continuously needed. GABAA receptors have diverse modulating binding sites and broad heterogeneity and are the main targets of prescribed sleep medicines such as benzodiazepines, barbiturates, and neurosteroids [11]. Recording electroencephalography (EEG) in animals is the most important technique for revealing the sleep architectures of hypnotics [12, 13]. Further, the sleep-enhancing effects of various treatments have been investigated using an EEG study in rodents [14]. Sleep is composed of two principal phases in mammals: rapid eye movement (REM) sleep and non-REM (NREM) sleep. REM sleep is characterized by fast waves with muscle atonia, brain activation, and eye movement, whereas NREM sleep is characterized by slower frequency delta waves [15]. It is also important to understand the abilities of Poria cocos ethanol extract (PCE) and PA to alter sleep architectures since sleep disorders can be caused by changes in REM and NREM sleep.
Sedative-hypnotics are commonly used to treat insomnia and modulate anxiety levels by targeting GABAA receptors, which are the primary mediators of fast inhibitory neurotransmission in the central nervous system (CNS) [16]. These receptors, which modulate the function of chloride ion channels, are activated by the inhibitory neurotransmitter GABA. A large family of constituent subunits (α1-6, β1-3, γ1-3, δ, ρ1-3, θ, π and ε) are present among various GABAA receptor subtypes [17]. Herbs such as valerian (Valeriana officinalis), St. John’s wort (Hypericum perforatum), passion flower (Passiflora incarnata), hops (Humulus lupulus), and kava kava (Piper methysticum) are often used for treatment of mild insomnia in Western societies [18]. These herbs have been found to exert sedative-hypnotic effects by regulating neurotransmitters such as γ-aminobutyric acid (GABA)-ergic or serotonergic systems in the CNS [19]. In addition, compounds extracted from these medicinal plants are of great importance in treatment of insomnia [20].
The aim of this study was to determine whether or not PCE and PA could serve as potential sedative/hypnotic agents to induce sleep. Unlike previous studies, this report focuses on whether or not PCE and PA could modulate sleep architectures in rats. Furthermore, intracellular chloride influx and over-expression of GABAA-receptors were measured to understand possible mechanisms and to obtain useful information for treatment of insomnia.
Materials and Methods
Poria cocos was purchased from Gyeongdong Herbal Market (Seoul, Korea). The sample was authenticated by Professor Mi Kyeong Lee, who works at the Laboratory of Pharmacognosy, Chungbuk National University. The voucher specimen (CBNU2013-PC) was kept in the herbarium of the department. Samples were piled up on the ground and air-dried. The air-dried sample (500 g) was extracted with 50% ethanol three times at room temperature. Extract was concentrated under pressure to yield a brown crude extract, which was centrifuged, filtered, and concentrated in a vacuum using a rotary evaporator to yield dried powder (100 g).
Pachymic acid (Fig. 1, purity HPLC, 98%) was purchased from Chengdu Biopurify Phytochemicals Ltd. (Chengdu, Sichuan, China). Dimethyl sulfoxide (DMSO) was purchased from Amresco (Solon, Ohio, USA) and muscimol from Tocris Bioscience (Bristol, UK). Pentobarbital sodium and diazepam were obtained from Hanlim Pharm. (Seoul, Korea) and Samjin Pharm. (Seoul, Korea), respectively. Fetal bovine serum (FBS) and Dulbecco’s Modified Eagle Medium (DMEM) were obtained from GIBCO (Grand Island, NY, USA). The Cl– sensitive fluorescence probe N-(ethoxycarbonyl-methyl)-6-methoxyquinolinium bromide (MQAE) was purchased from Sigma-Aldrich (St Louis, MO, USA). Specific rabbit polyclonal antibodies against GABAA receptors subunits or GAD65/67 as well as corresponding conjugated anti-rabbit immunoglobulin G-horseradish peroxidase were obtained from Abcam (Cambridge, UK). Chemiluminescent horseradish peroxidase substrate was purchased from Millipore (Billerica, MA, USA).
Male Sprague-Dawley rats weighing 250~280 g each were purchased from Samtako (Osan, Korea). Each animal was housed individually in acrylic cages (45 × 60 × 23 cm) with water and food available ad libitum under an artificial 12-hr light/dark cycle (light on at 7:00 a.m./off at 7 p.m.) in a room with a constant relative humidity (50~52%) and temperature (22 ± 2°C). Animals were kept in the departmental holding room 1 week before testing. This study was performed in accordance with the National Institute of Health Guide for Care and Use of Laboratory Animals (NIH publication No. 85-23, revised 1985), and the Institutional Animal Care and Use Committee of Chungbuk National University approved the protocol.
After a minimum 7-day acclimation period, each rat was implanted with a transmitter (TL10M3-F50-EEE; Data Sciences International, St. Paul, MN, USA) for recording EEG and activity via telemetry as previously described [21]. The body of the transmitter was subcutaneously implanted off the midline and posterior to the scapula, followed by attachment to the skin with three sutures for stabilization. Transmitters led subcutaneously (s.c.) to the skull, and bare ends were placed in contact with the dura through holes in the skull (A: 2.0 [Bregma], L: 1.5; P: 7.0 [Bregma], L: 1.5 contra-lateral) [22]. The electrodes were anchored to the skull with screws and dental cement. All surgical procedures were performed stereotaxically under aseptic conditions. Surgical anesthesia was achieved with pentobarbital (50 mg/kg, i.p), and surgical procedures were made to minimize animal suffering. Telemetric recording of cortical EEG and activity was conducted using procedures similar to previous reports. For the EEG signal, transmitter gain was set at – 0.5/+ 0.5 volts per/units × 2, and raw signals generated from the transmitter were in the range of 0.5~20.0 Hz. Signals were processed using a Data Sciences International analog converter and routed to an AD converter (Eagle, PC30, USA) housed in a personal computer (PC). An AD converter was used to digitize the EEG and activity signals at 128 Hz. Digitized data were transferred to the computer and displayed graphically. Online fast Fourier transformation (FFT) was performed on the EEG data at 10-sec intervals during data acquisition (1,024 samples) after Hanning window treatment. FFT analysis generated power density values from 0 to 20 Hz at a resolution of 0.5 Hz. FFT data were further averaged in the range of 0 to 20 Hz every 10 sec. Sleep data and FFT results were saved to hard disk every 10 sec for additional offline analysis. Movement of the animal in relation to the telemetry receiver generated transistor-transistor logic (TTL) pulses, which were collected and counted as a measure of activity. PA was suspended and dissolved in 0.1% DMSO, and PCE was dissolved in physiological saline. PCE (100 mg/kg) and PA (5 mg/kg) were administered orally to rats 1 hr prior to the recording. Animals were allowed to habituate to a polygraphic recording environment in which they could freely move. Then, polygraphic signs of sleep-wake activities were recorded for 6 hr starting at 10:00 am.
Wakefulness, NREM, and REM sleep were determined from the digitized data at 10-sec intervals using sleep analysis software, SleepSign 2.1 (KISSEI Comtec, Matsumoto, Japan). This software identifies wakefulness as a high-frequency, low-amplitude EEG and NREM based on the presence of spindles with slow waves. EEG power during REM is significantly reduced lower frequency δ-waves (0.75~4.0 Hz) and increased θ-wave activity (5.0~9.0 Hz, peak at 7.5 Hz). Data from seven or eight rats in each group were analyzed. Time periods spent in NREM and REM, total sleep time (NREM + REM), and numbers of sleep-wake cycles were processed in a 6-hr period for each rat.
Hypothalamus neuronal cells in primary culture were prepared from 8-day old Sprague-Dawley rats as previously described [23]. These cells expressed functional GABAA receptors after being cultured for 8 days [24, 25]. Briefly, cells were plated (1.0 × 105 cells per 0.2 mL) in 96-well microplates or (5.0 × 105 cells per mL) 60-mm dishes coated with poly-L-lysine (50 μg/mL; Sigma-Aldrich, St. Louis, MO, USA), followed by culture in DMEM nutrient and neurobasal A media supplemented with 10% heat-inactivated fetal bovine serum, glutamine (2.0 mM), gentamicin (100 μg/mL), antibiotic-antimycotic solution (10 μg/mL; Sigma-Aldrich), and potassium chloride (25 mM). A high concentration of potassium was necessary to induce persistent depolarization, which promotes survival of granule cells. Cells were incubated for 6~9 days in a humidified 5% CO2/95% air atmosphere at 37°C. Cytosine arabinofuranoside (final concentration, 10 μM; Sigma-Aldrich) was added to cultures for 18~24 hr after plating to inhibit proliferation of non-neuronal cells.
Intracellular Cl– concentration ([Cl–]i) of rat hypothalamic neurons was estimated using the Cl–-sensitive fluorescence probe MQAE with slight modification of a previously described method [26]. Buffer (pH 7.4) contained 2.4 mM HPO42–, 0.6 mM H2PO4–, 10 mM HEPES, 10 mM D-glucose, and 1 mM MgSO4. A variety of MQAE-loading conditions were assessed. Cells were incubated overnight in medium containing 10 mM MQAE. After loading, cells were washed three times in appropriate Cl–-containing or Cl–-free buffer. The buffer was replaced with buffer or without the compounds or Cl– free buffer. Repetitive fluorescence measurements were conducted immediately using a SpectraMax M2e Multi-Mode Microplate Reader (excitation wavelength: 320 nm, emission wavelength: 460 nm; Molecular Devices, Downingtown, PA, USA). Data are presented as the relative fluorescence F/F0, where F is the fluorescence as a function of each sample and F0 is the fluorescence without Cl– ions. The F/F0 values were directly proportional to [Cl–]i.
Primary rat hypothalamic neuronal cells were cultured for 8 days. PCE was dissolved in 0.9% physiological saline and diluted sequentially in culture medium to final concentrations of 50 and 100 μg/mL. Cells were treated for 1 hr. The control group was treated with vehicle at the same dilution used for drug treatment. Culture medium was completely replaced with fresh medium containing the appropriate drug. Cells were harvested and treated with lysis buffer. Extracts were centrifuged at 13,000 × g at 4°C for 10 min, and the supernatant was recovered. Protein concentration of the supernatant was determined and stored at 20°C. Total protein concentration was determined by the modified Lowry method using bovine serum albumin as a standard [27].
For Western blot analysis, an equal amount of protein was added to each lane, and sodium dodecyl sulfate polyacrylamide gel electrophoresis (SDS-PAGE) was performed using 10% polyacrylamide gels. Proteins were transferred to polyvinylidene difluoride (PVDF) membranes (Hybond-P, GE Healthcare, Amersham, UK) using a semidry transfer system. Blots were blocked for 1 hr at room temperature with 5% (w/v) BSA and incubated with primary antibodies. To detect glyceraldehyde-3-phosphate dehydrogenase (GADPH), blots were blocked with for 1 hr at room temperature with 5% (w/v) skim milk in Tris-buffered saline solution (TBS) containing 0.1% Tween-20. The membrane was incubated with specific rabbit polyclonal antibodies against GABAA receptors subunits (diluted 1:2,500 in TBS containing 0.1% Tween-20, 5.0% BSA) and rabbit anti-GAD65/67 polyclonal antibody (diluted 1:2,500 in TBS containing 0.1% Tween-20, 5.0% BSA). Blots were then washed and incubated with horseradish peroxidase conjugated secondary antibody: goat anti-rabbit IgG (diluted 1:3,000 in TBS containing 0.1% Tween-20, 1.0% BSA). Immunoreactive bands were developed with a BM chemiluminescence detection kit (Roche Diagnostics, Mannheim, Germany). Quantitative analysis of detected bands was performed with densitometric scanning, and all values were normalized to the amount of GAPDH in the sample, which was measured as follows. All immunoblots were stripped, incubated with sheep anti-GAPDH antibody (1:2,500 in TBS containing 0.1% Tween-20), subsequently incubated with anti-sheep IgG-conjugated secondary antibodies, rabbit anti-sheep IgG (1:3,000 in TBS containing 0.1% Tween-20), and developed to confirm equal protein loading.
All statistical analyses were conducted using SigmaStat software (SPSS, Chicago, IL, USA). Data are expressed as mean ± S.E.M. The significance of the compounds’ effects was assessed by analysis of variance (ANOVA). Significant differences after one-way ANOVAs were measured by post hoc Holm-Sidak test. A P-value<0.05 was considered to be significant.
Results
PCE (100 mg/kg) and PA (5 mg/kg) were orally administered to measure sleep architectures by EEG. PCE (100 mg/kg) and PA (5 mg/kg) caused significant reduction in the number of sleep-wake cycles over a 6-hr period (Figs. 2A and 2C). PA increased NREM, REM, and total sleep times and decreased wakefulness (Fig. 2B). Moreover, PCE significantly increased NREM and total sleep times compared to the control group (Fig. 2D).
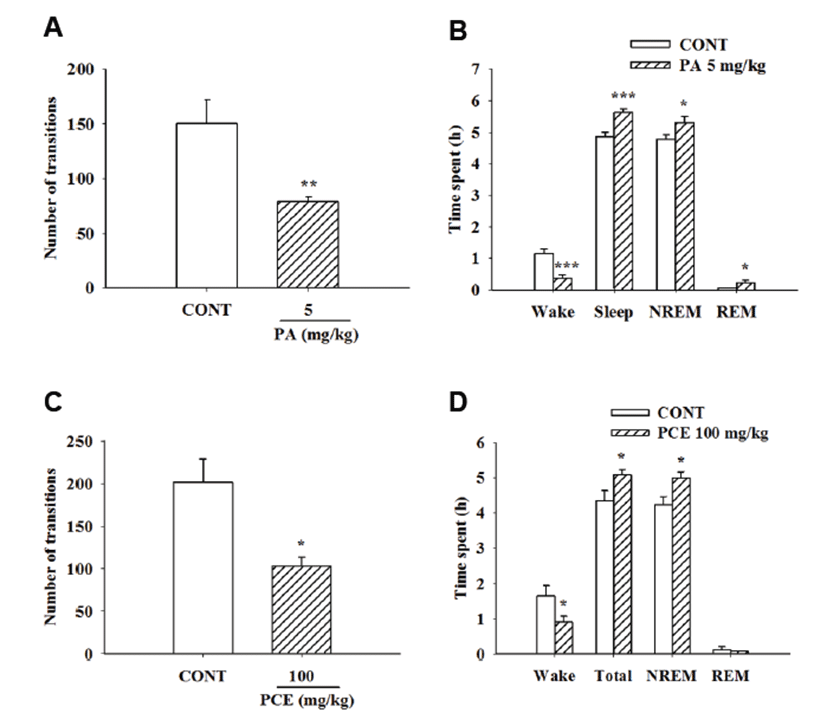
PCE (0.1, 1.0, and 10 μg/mL) significantly increased influx of chloride ion in primary cultured neuronal cells in a concentration-dependent manner. In addition, pentobarbital (PENT, 10 μM) was used as a positive control and significantly increased influx of Cl– in cultured cells (Fig. 3).
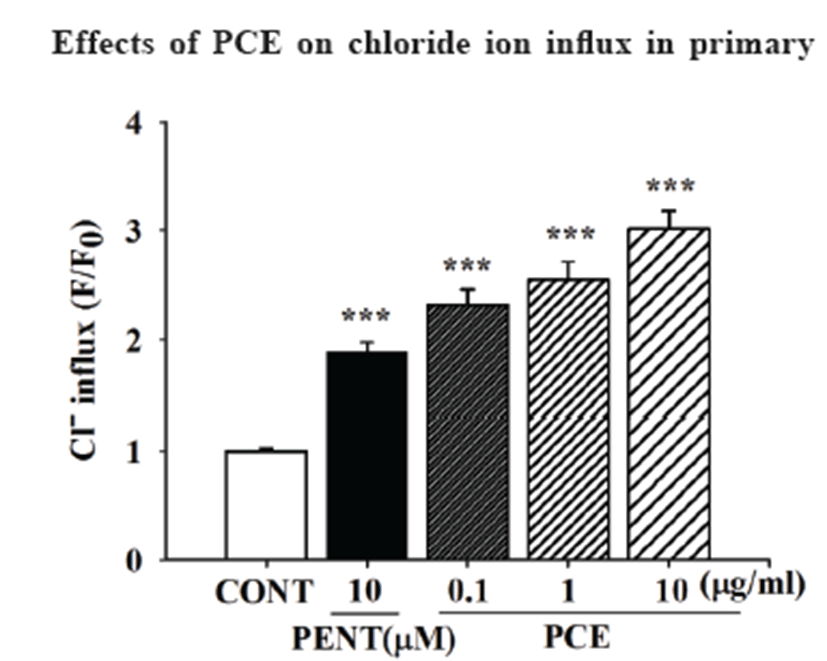
To assess whether or not PCE enhances sleeping behaviors via biosynthesis of GABA, primary cultured hypothalamic neuronal cells were treated with PCE (50 and 100 μg/mL) to examine activation of GAD65/67. PCE significantly increased protein content of GAD65/67 (Fig. 4).
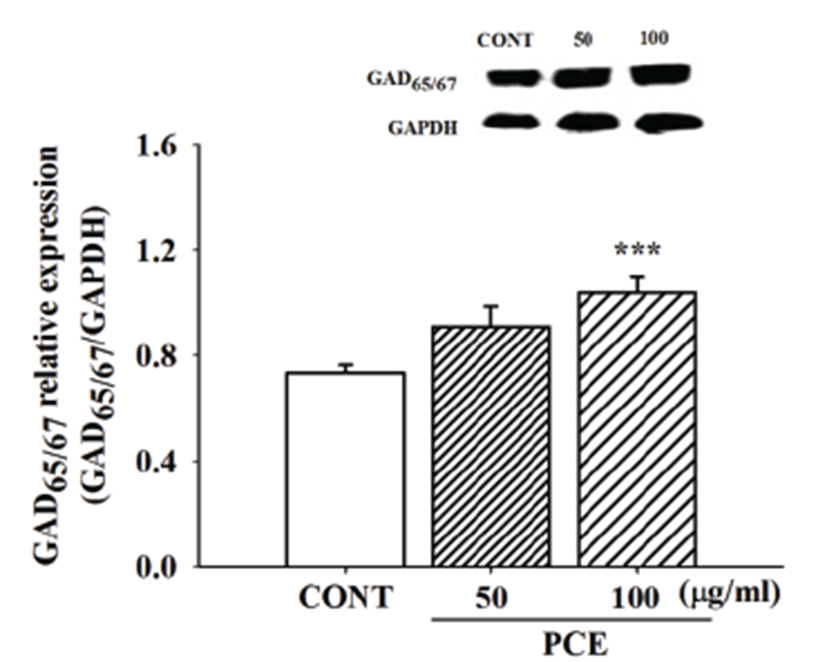
Moreover, to assess whether or not PCE activates GABAA receptor subtypes, primary cultured hypothalamic neuronal cells were treated with PCE (100 μg/mL), and Western blot analysis was used to measure expression levels of GABAA receptor subunits. GABAA receptor α-,β-, and γ-subunits were overexpressed after cells were treated with PCE. Additionally, pentobarbital (10 μM) was used as a positive control and led to over-expression of protein levels of GABAA-receptor subunits (Fig. 5).
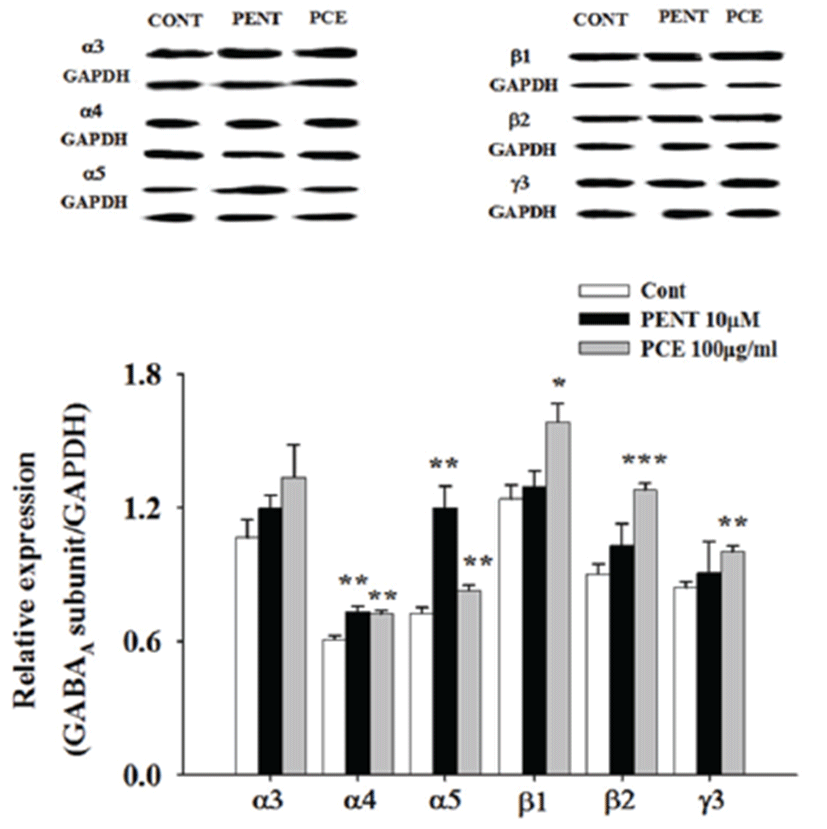
Discussion
Poria cocos is a well-known traditional Chinese herb that has long been used in Asian countries to treat a range of sleep disorders, such as insomnia, as either a single herb or part of an herbal formula [2]. Recently, PA, a main component of PCE, was shown to increase pentobarbital-induced sleeping behaviors via GABAA-ergic systems [9]. Researchers suggest that the inhibitory neurotransmitter GABA is involved in generation of various brain rhythmic activities, which can be modulated by benzodiazepines. The ultimate goal of this study was to evaluate whether or not PCE and its active constituent, PA, modulate sleep architectures via activation of GABAA-ergic transmission in rodents.
Spectral EEG frequency analysis is a useful analytical technique for differentiating sleep/wake state changes for sleep-inducing insomnia treatments. Sleep can be divided into two major stages: REM and NREM sleep. During the early years of sleep research, NREM sleep was discovered to play a role in the restoration of physiological functions [28]. REM sleep is characterized by fast wave sleep along with muscle atonia, brain activation, and eye movement. We especially focused on determining whether or not PCE and its active component, PA, increase sleep time and alter sleep architectures such as REM and NREM sleep. Our experimental data show that both PCE and PA caused significant reduction in the number of sleep-wake cycles.
Furthermore, we determined whether or not PCE may alter sleep architectures. PCE oral administration noticeably enhanced NREM and total sleep times and decreased wakefulness and REM sleep. The present data confirm that PCE increases total sleeping time and NREM sleep. Interestingly, PA increased NREM, REM, and total sleep times and decreased wakefulness in rodents based on analysis of EEG recording. Thus, it is important that both PCE and PA increase sleeping behaviors via GABAA-ergic mechanisms in the CNS [9]. In terms of herbal insomnia treatments, research has revealed that Ganoderma lucidum prolongs sleep time in rats in a similar fashion [14]. PCE and PA are used for their somnogenic effects. Previous research revealed that orexin receptor antagonists (DORAs) promote sleep that is more similar to physiological sleep and quantitatively different from that induced by GABAA modulators [29].
The present study focused on whether or not prolonged sleeping behaviors by PCE are mediated by GABA-ergic systems. Emerging evidence indicates that GABAA receptors play an important role in the modulation of barbiturate-induced sleep through the GABAA-ergic system [30]. Increased pentobarbital-induced sleep time can be a useful tool for examining inhibitory effects on the CNS, and especially for investigating effects on GABAA-ergic systems in the CNS [23, 31]. Of note, GABAA modulators have been associated with CNS-related adverse events (AEs), including sleep walking, sleep driving, sleep eating, amnesia, and cognitive impairment [32-35]. Moreover, natural products and their derived comcompounds have been shown to offer neuroprotective and anti-inflammatory activities [36, 37]. In addition, our study investigated whether or not PCE triggers opening of GABA receptor-coupled Cl– channels of GABAA receptors. Treatment of hypothalamic neuronal cells with PCE and pentobarbital significantly increased influx of Cl–in this culture model. These effects indicate that benzodiazepines act by allosterically binding to GABAA receptors and enhance the ability of GABA to increase chloride conductance.
GABA is synthesized from glutamate exclusively in GABA-ergic neurons by glutamic acid decarboxylase (GAD), which consists of two isoforms with molecular weights of 65-kDa and 67-kDa and catalyzes formation of GABA from glutamic acid [38, 39]. Protein expression levels of GAD65/67 were measured in primary cultured hypothalamic neuronal cells. PCE increased protein content of GAD65/67 in these cells. Expression studies have indicated that subunit composition determines the GABA sensitivity and pharmacological properties of GABAAreceptors [40-42]. GABAA receptors play an important role in neuronal firing patterns and activity of neuronal networks. Furthermore, they serve as targets for numerous classes of drugs and are used both in clinical practice and as research tools.
GABAA receptors are important therapeutic targets for treatment of insomnia since they rapidly inhibit neurotransmission and participate in tonic inhibition [43]. Structural and physiological heterogeneities of the pentameric composition of GABAA receptors as well as the differential distribution of its receptors subtypes in specific brain areas provide an important basis for the development of therapeutic drugs. GABAA receptors with a classic high-affinity benzodiazepine binding site contain α-, β-, and γ-subunit combinations. Benzodiazepines act as facilitators of fast inhibitory neurotransmission in the mammalian brain mediated through GABAA receptors, which are involved in the regulation of vigilance, anxiety, muscle tension, epileptogenic activity, and memory functions [44]. We examined expression patterns of α-subunits (α3, α4, α5), β-subunits (β1, β2) and γ-subunit (γ3) in GABAA-receptors in primary cultured cerebellar granule cells. Qualitative results from Western blot analysis show that both PCE and pentobarbital treatment induced over-expression of GABAA receptors subunits. Moreover, PCE significantly increased protein level content in α4, α5, β1, β2, and γ3 subunits.
Over the last decade, scientists have shown increased interest in herbal medicines, which contain phytochemical constituents with long-term health promoting or medicinal qualities as well as anxiolytic and sedative properties. Ethanol extract of Fructus Schisandrae fruit [45] and Gomisin N isolated from Schisandra chinensis were shown to have beneficial sedative and hypnotic bioactivities, which supports their ability to treat insomnia [46]. Pharmacological treatments for insomnia may be required to reduce sleep latency, increase sleep maintenance, and improve sleep quality in order to successfully manage insomnia.
In summary, the present study provides evidence that PCE and PA possess not only a sleep-prolonging effects but also sleep quality-enhancing effects when administered orally to rats. Activation of GABAA-ergic transmission plays an important role in sleep promotion. These results also provide a pharmacological basis for the therapeutic efficacy of PCE and its active component, PA, in treatment of insomnia. However, further experiments are needed to better elucidate the pharmacological effects of other compounds derived from PCE.