Introduction
Peripheral arterial disease (PAD) is a common disease found in 10~25% of patients over the age of 55 years and krits severity and occurrence are strongly associated with other cardiovascular risk factors that lead to coronary artery disease and stroke [1]. It is associated with diminution in quality of life and increased mortality. However, interventional or surgical procedures are not available in some conditions due to the complexity of the disease. Alternative therapy includes the attempt to develop new collateral vessels using angiogenic growth factors. However, the optimal therapeutic approach has not been developed [2].
Angiogenesis, the formation of new blood microvessels from preexisting ones, in adult organisms is normally limited to the female reproductive system. However, the formation of new blood vessels becomes essential during wound healing and tissue repair and pathological conditions [3].
Recently, activators of the endothelial nitric oxide (eNOS)/nitric oxide (NO) pathway such as vascular endothelial growth factor (VEGF) have been reported to promote angiogenesis or arteriogenesis in critical limb ischemia [4]. The regulation of angiogenesis by hypoxia is an important component of homeostatic control. The major factor which responds to hypoxia is hypoxia-inducible factor-1α (HIF-1α). It modulates transcriptional control of several genes such as VEGF and eNOS [5].
Thymosin β4 (Tβ4) is a highly conserved, 43-amino acid acidic peptide that was first isolated from calf thymus tissue. The most prominent physiological function of Tβ4 is the regulation of actin polymerization which is necessary for cell motility and organogenesis [6]. Recently, the healing properties of Tβ4 have been found in the skin [7, 8] and cornea [9, 10], and more recently it has been shown that Tβ4 facilitates cardiac repair after infarction by promoting cell migration and myocyte survival [11, 12]. And Tβ4 promotes vasculogenesis and angiogenesis [13, 14] after ischemic injury. Safety, toler-ability and efficiency of Tβ4 were evaluated in clinical patients with acute myocardial infarction [6] and other diseases [9, 10, 15].
We hypothesized that recombinant Tβ4 (rTβ4) may be involved in the mechanisms of angiogenic pathways and formation of collateral vessel after PAD, resulting in the promotion of angiogenesis. Our study reveals the effects of rTβ4 on peripheral angiogenesis in a mouse hindlimb ischemia model and rTβ4 may contribute to the advancement of alternative therapeutic medicine in this field.
Materials and Methods
Specific pathogen-free 11-week-old male C57BL/6 mice were purchased from a commercial animal breeder (Koatech Inc., Pyeongtaek, Korea) and acclimated for 1 week before initiation of the study. During the acclimation and experimental periods, the mice were housed in polycarbonate cages in an animal room with controlled temperature (22 ± 3°C) and humidity (50 ± 20%) and a 12-hr light/dark cycle. The animals were provided commercial pellets (Purina, Seoul, Korea) and tap water ad libitum. All procedures were conducted incompliance to the Standard Operating Procedures of Laboratory Animals and approved by Laboratory Animal Care and Use Committee of the Laboratory Animal Research Center, Chungbuk National University. rTβ4 was obtained from Biotoxtech Co., Ltd. (Ochang, Korea). Sterile saline was obtained from Choongwae Pharma Co., Ltd. (Seoul, Korea) and used as the vehicle.
Mice were anesthetized with intramuscular injection of Zoletil (30 mg/kg) and xylazine (8 mg/kg). Aseptic surgery was performed to ligate the right common femoral artery. In brief, an incision was made in right groin of mice. Blunt dissection was performed and the right common femoral artery was identified by its pale pink color and pulsatile nature. The common femoral vein and femoral nerve were dissected free of the artery. Two ligations were performed in the common femoral artery proximal to the origin of the profundafemoris artery using 7-0 nylon sutures. The common femoral artery was then transected between the ligation sites. Immediate blanching was noted in the distal right hind limb after ligation. The incision was sutured in a single layer using the same suture material as for ligation. Mice were noted to be limping and dragging the right hind limb after recovery from anesthesia [16].
Sixteen operated mice received rTβ4 immediately after ligation and the remaining 6 animals received saline alone. Treatment was given intramuscularly (IM) or intraperitoneally (IP) with rTβ4 (150 μg in 300 μL saline/mouse, n=8). Injections were given every 3 days during 3 weeks [11, 17] (Fig. 1). Dosages were based on previous studies on Tβ4 biodistribution [18].
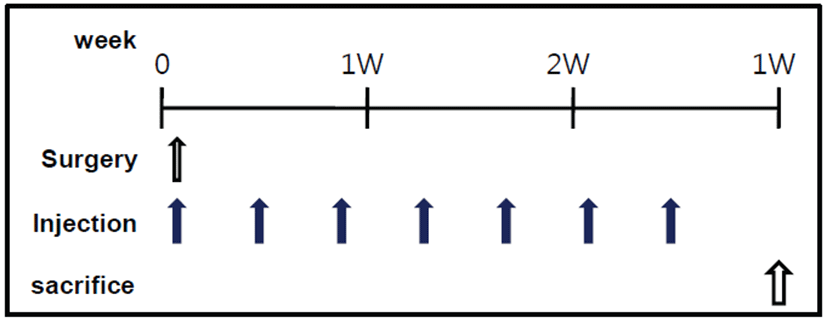
Right hindlimbs were removed, weighed, and fixed for histological sectioning. The quadriceps femoris muscles were excised and fixed in 4% paraformaldehyde solution. Some muscle samples were routinely processed, paraffin-embedded, cut into 4-μm sections, and stained with Masson’s trichrome.
Remaining samples were used for immunohistochemical study. CD31, HIF-1α, VEGF-1 and eNOS immunoreactivities were identified using monoclonal anti-CD31 antibody at 1:100 (M0823) (Dako Biotechnology, Carpinteria, CA, USA), monoclonal anti-HIF-1α antibody at 1:100 (sc-53546) (Santa Cruz Biotechnology, Santa Cruz, CA, USA), monoclonal anti-VEGF-1 antibody at 1:100 (sc-53462) (Santa Cruz Biotechnology) and monoclonal anti-eNOS antibody at 1:100 (sc-654) (Santa Cruz Biotechnology). The reaction was visualized with ABC reagent (Santa Cruz Biotechnology). Briefly, the sections were incubated in 3% hydrogen peroxide to block endogenous peroxidase activity. The slides were treated with antigen retrieval solution (0.1% trypsin) at 37°C for 20 min. To prevent nonspecific antibody binding, sections were preincubated for 30 min in PBS containing 10% goat serum. The sections were incubated with the primary antibodies directed against CD31, HIF-1α, VEGF-1 or eNOS at appropriate dilutions 2 hr at 37°C and then rinsed for 15 min with PBS followed by incubation with secondary antibody (rabbit monoclonal to mouse IgG, ABC kit) for 1 hr at 37°C. Again the sections were rinsed with PBS and incubated with diaminobenzidine and counterstained with hematoxylin. Negative control slides were prepared by substituting PBS for CD31, HIF-1α, VEGF-1 and eNOS staining.
Whole muscle cell lysates were fractionated by SDS-PAGE and transferred onto membranes (Millipore Corp., Billerica, MA, USA). Monoclonal antibodies against HIF-1α (Santa Cruz Biotechnology), VEGF-1 (Santa Cruz Biotechnology) and eNOS (Santa Cruz Biotechnology) were diluted at 1:500 and β-actin (Fermentas Biotechnology, Burlington, Ont, Canada) was diluted at 1:2,000. Each antibody was used in conjunction with a horse radish peroxidase-conjugated secondary antibody (Santa Cruz Biotechnology).
The differences between the groups were examined using a standard one-way analysis of variance. If statistical significance differences were evident, the data were analyzed using the multiple comparison procedures of Duncan’s or Dunnett’s multiple range comparison. SPSS for Windows 12.0 K software package was used.
Results
Mice were noted to be limping and dragging the right hindlimb after surgery. To evaluate the ischemic injury and distribution of collateral vessels in the right hindlimb muscular tissue, right hindlimb without skin was observed by stereoscopic microscope at the end of the experiment. The ischemia control group exhibited marked ischemia-induced muscular atrophy compared to the normal control (Figs. 2A and 2B). However, there were no significant differences between the treatment groups with rTβ4 (IM and IP) and the ischemia control group in the ratio of right to left hindlimb (Fig. 3).
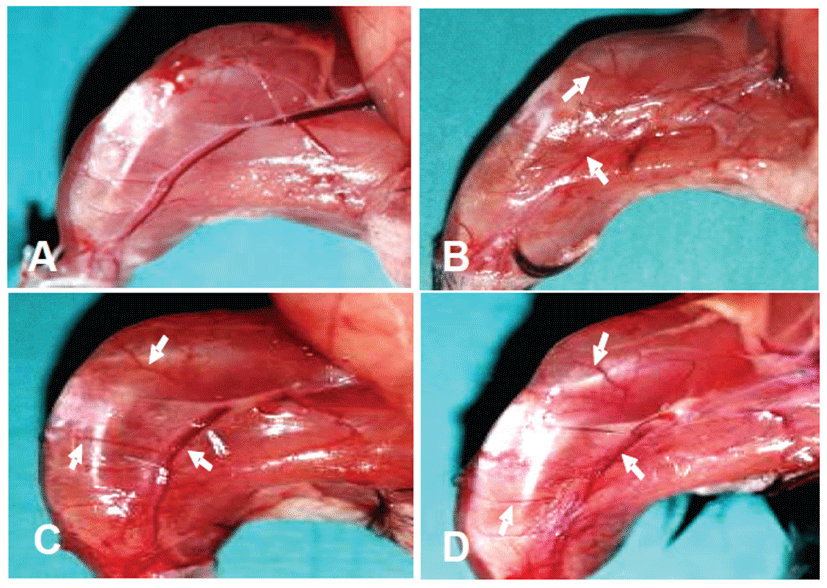
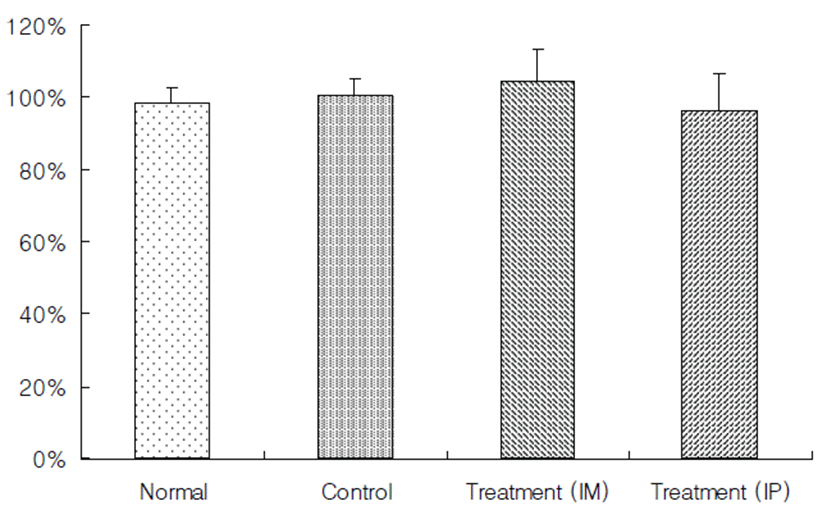
In the distribution of collateral vessels, treatment groups with rTβ4 induced more number of collateral vessel in comparison to the ischemia control group (Figs. 2B, 2C and 2D).
To investigate fibrotic changes in the hindlimb, Masson’s trichrome stain was used. The ischemia control exhibited fibrous connective tissue components around the endomysium and intramuscular area caused by muscular dystrophy compared to the normal control (Figs. 4A and 4B). However, treatment groups with rTβ4 (IM and IP) showed diminished fibrosis in both the perimysiym and endomysium area (Figs. 4C and 4D).
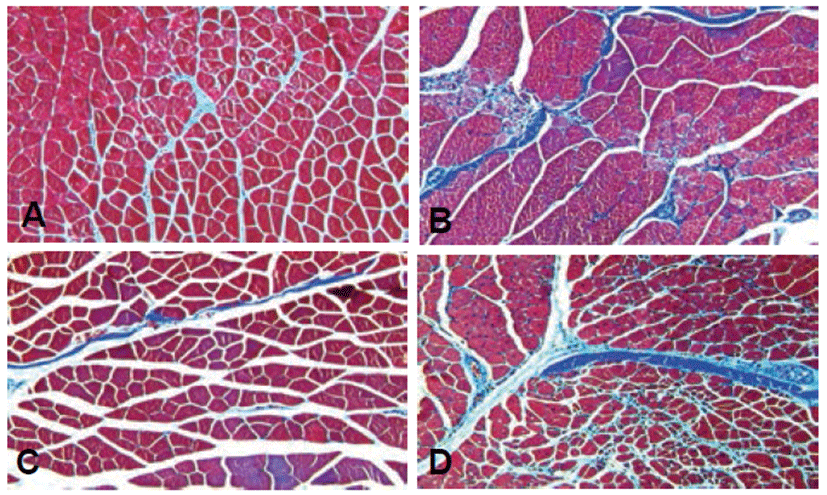
To assess the formation of microvessels, CD31 immunostaining was used. CD31 in the ischemic control group was observed to be similarly expressed compared with the normal control group. While the treatment group compared with the ischemia control group as a whole showed a high expression of CD31. These results suggest that the expression of CD31 is considered to be one to stimulate microvascular formation. As a result, rTβ4 treatment group compared with the ischemia control group showed high CD31 expression patterns (Fig. 5).
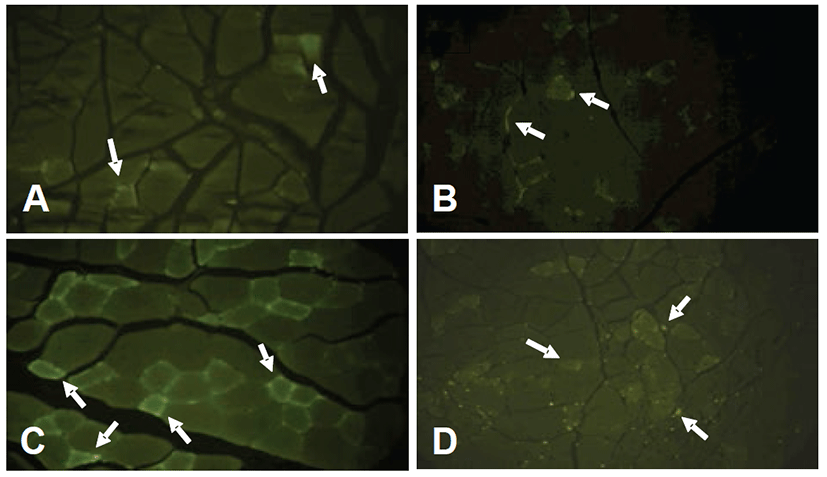
Also, the expression of HIF-1α exhibited similarly in the ischemic control group compared to the normal control group, while the expression of HIF-1α increased in the treatment group compared to the ischemic control group (Fig. 6). In the immunohistochemical staining for VEGF-1 and eNOS, similar patterns were observed as the HIF-1α (Figs. 7 and 8).
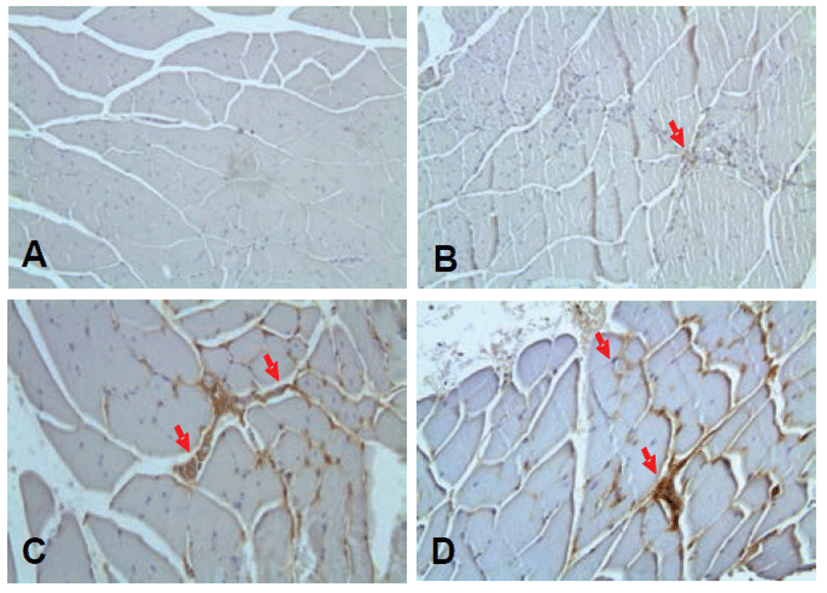
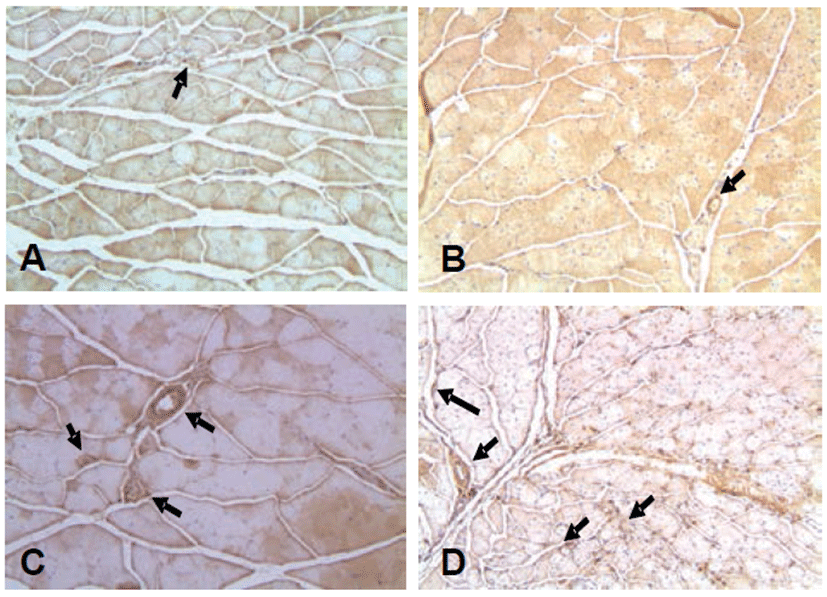
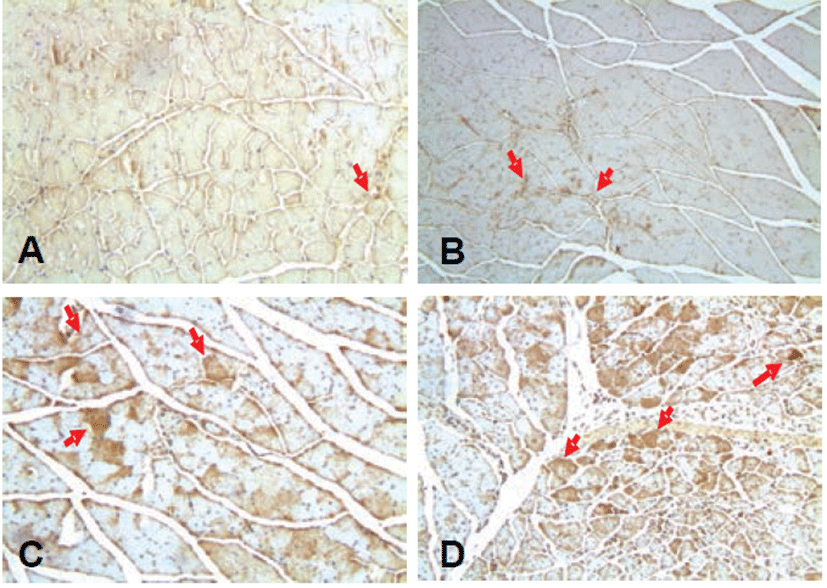
The expressions of HIF-1α, VEGF-1 and eNOS were enhanced in the treatment groups with rTβ4 (IM and IP) by Western blot analysis. The expression of HIF-1α, VEGF-1 and eNOS was up-regulated in treatment groups with rTβ4 as compared with the ischemia control (Fig. 9).
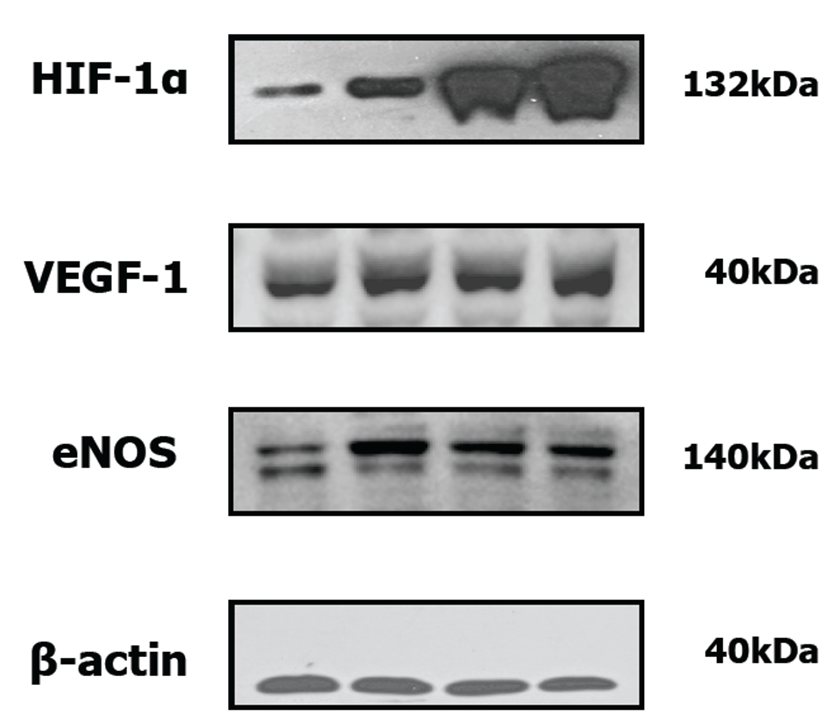
Discussion
The effects of rTβ4 was tested on angiogenesis in a mouse hindlimb ischemia model. During 3 weeks, rTβ4 was administered every 3 days. To assess the recovery of ischemic tissue damage and the expression of angiogenic growth factors in an ischemic hindlimb, histopathological analysis and Western blotting were performed. Data was presented the alleviation of ischemic tissue damage and the overexpression of angiogenic growth factors.
Ischemia can cause ischemic damage to hindlimb muscle and eventually lead to tissue necrosis [2]. In gross findings, the ischemia controls displayed muscular atrophy. Previous studies showed muscular atrophy by ischemia compared to the nomal control, leading to decreased ratio of left to right leg volume [19, 20]. However, there were no significant differences between the treatment groups with rTβ4 (IM and IP) and the ischemia control in the present study. According to the study by Hoefer et al (2001), there was no difference between treatment groups and ischemia control when the treatment was performed 3 weeks after fermoral ligation. Additional experiments to evaluate the ratio of ischemic to non-ischemic hindlimb by date are needed. To investigate fibrotic changes in the ischemic hindlimb, Masson’s trichrome staining was used. Microscopic analysis revealed the ischemia control exhibited fibrous connective tissue components around endomysium and intramuscular area caused by muscular dystrophy. Treatment groups with rTβ4 (IM and IP) showed diminished fibrosis in both the perimysium and endomysium area. These data indicated that rTβ4 reduced the ischemic injury and prevented the muscular dystrophy.
Ischemia-induced hypoxia stimulates the release of HIF-1α. It modulates transcriptional control of VEGF-1 and eNOS. NO, produced by eNOS, is an important regulator of angiogenesis and can induce angiogenesis in vivo [21]. rTβ4 induced angiogenesis via eNOS using thermal theraphy in mice with hindlimb ischemia [17]. To explain the mechanism of rTβ4, the expression of angiogenic growth factors was evaluated by Western blotting. Data revealed that the expression of HIF-1α, VEGF-1 and eNOS enhanced in the treatment groups with rTβ4 (IM and IP) compared to the ischemia control. Also, the histological analysis showed that the expression of HIF-1α, VEGF-1 and eNOS increased in the treatment groups with rTβ4 (IM and IP) compared to the ischemia control in ischemic hindlimb muscle. These results suggest that rTβ4 promotes the expression of angiogenic growth factors related to the NO/eNOS pathway.
Angiogenesis is the postnatal process whereby an existing microvascular network is expanded [3]. In angiogenesis, collateral vessels were observed [22]. Although we could not perform an angiography, we speculated the formation of blood microvessels by CD31 immunostaining. CD31 immunostaining was widely used in detecting new blood microvessels and expression of CD31 increased depending on number of new blood microvessels [23]. The treatment groups with rTβ4 (IM and IP) induced the over-expression of CD31 compared to the ischemia control. This study found an increase in the expression of angiogenic growth factors after administration of rTβ4 in a mouse hindlimb ischemia model. Therefore, it is assumed that rTβ4 was involved in the angiogenesis and formation of collateral vessels.
In conclusion, the results indicate that rTβ4 exerts angiogenic properties through increased angiogenic growth factor expression and that rTβ4 is likely to be developed as an alternative therapeutic medicine in peripheral arterial disease.