INTRODUCTION
Saliva is essential for digestion, taste, cleansing, and antimicrobial action. Salivary gland dysfunction can be caused by various factors such as systemic diseases, medications, radiation, and aging [1-3]. Diabetes has long been known to be associated with oral diseases [4, 5]. Oral dryness is a common symptom in diabetic patients, and changes in the salivary glands and their functions are linked to hyperglycemia Patients with diabetes report symptoms of dry mouth and decreased salivary secretion [6-9]. Inadequate saliva production can damage tissue, making it more susceptible to infections [10]. Current treatments for impaired salivary secretion offer only temporary relief and fail to address the underlying cause. Therefore, research into the mechanisms and treatments of salivary gland dysfunction is necessary to improve salivary gland function [11, 12].
Glucagon-like peptide 1 (GLP-1) is the major incretin hormone released from L cells that regulates blood sugar by stimulating glucose-dependent insulin secretion and suppressing glucagon secretion [13, 14]. Dipeptidyl peptidase-4 (DPP-4) is an enzyme responsible for breaking down GLP-1. DPP-4 inhibitors, such as sitagliptin, vildagliptin, and saxagliptin, prevent the inactivation of endogenously released incretin hormones, prolonging their physiological actions. DPP-4 inhibitors have roles beyond glucose regulation; they have cytoprotective effects against various organ damages DPP-4 inhibitors have demonstrated protective effects against various diabetes complications, including non-alcoholic fatty liver disease, impaired lipid function due to obesity, pancreatic beta cell atrophy, cardiovascular complications, and neuropathy [15]. Saxagliptin suppressed the increased insulin signaling impairment and oxidative stress in a D-galactose-induced aging model [16]. Linagliptin treatment improves diabetic retinopathy by reducing oxidative stress and decreasing the formation of advanced glycation end products (AGEs) [17]. Linagliptin also reduced AGE-mediated oxidative stress in cultured endothelial cells and lowered AGE levels, the expression of the receptor for AGE (RAGE), and oxidative stress in diabetic rats [18]. In animal studies, Vildagliptin prevented diabetes-related vascular damage by reducing AGEs, RAGE, and oxidative marker levels [19]. Recently, the DPP-4 inhibitor, alogliptin, was found to block the AGE-RAGE axis and reduce albuminuria in patients with type 2 diabetes [20]. Moreover, gemigliptin improved salivary gland function in streptozotocin-induced diabetic rats by exerting antioxidant and antiapoptotic effects [21]. Building upon previous findings, this study aimed to verify the pathological roles of DPP-4 and GLP-1 in diabetes-induced salivary gland dysfunction.
MATERIALS AND METHODS
Seven male db/db type 2 diabetic mice and seven male wild-type C57BL/6 mice, five weeks of age, were supplied by Central Lab Animal (Seoul, Korea). The mice were then randomly divided into the C57BL/6 control group (n = 7) and the diabetic db/db model group (n = 7). The animals were housed in a temperature (20°C–25°C) and humidity-controlled (40%–70%) barrier system with a 12/12 hr light/dark cycle. After five weeks, salivary glands were isolated from each mouse at necropsy. The animal experiments were conducted following the procedures approved by the Institutional Animal Care and Use Committee of Jeonbuk National University Laboratory Animal Center (IACUC, Approved No.: JBNU 2021-087).
The mice were anesthetized, and pilocarpine hydrochloride (1.5 mg/kg, IP) was administered. We then placed cotton balls in the mouths of the mice for 15 minutes to collect saliva. After 15 minutes, we measured the weight of the cotton balls to determine the amount of saliva absorbed. We compared and analyzed salivary secretion by calculating the difference in weight between the cotton balls before and after saliva absorption.
We conducted cell apoptosis analysis within the salivary gland tissue of mice. The process involved fixing the samples with formaldehyde, dehydrating them, and embedding them in paraffin blocks. Subsequently, we sectioned the paraffin blocks to 4 μm. Next, we deparaffinized the tissue sections twice in xylene (5 minutes each) and rehydrated them in ethanol. The terminal deoxynucleotidyl transferase dUTP nick-end labeling (TUNEL) assay was performed according to the DeadEndTM Fluorometric TUNEL System instructions from Promega (Madison, WI, USA). Cell nuclei were counterstained with DAPI mounting solution (4',6-diamidino-2-phenylindole, Santa Cruz Biotechnology, Santa Cruz, CA, USA), and TUNEL-positive cells were quantified. TUNEL-positive apoptotic cells were visualized with green fluorescence using a BX51 microscope (Olympus, Tokyo, Japan). Five high-power fields (× 200 magnification) were randomly selected for each group, and the number of TUNEL-positive cells was calculated using ImageJ software (NIH, Bethesda, MD, USA).
The sections, fixed in 10% formalin and embedded in paraffin, were deparaffinized and rehydrated. Hematoxylin and Eosin (H&E) staining was performed for histological evaluation. To assess the accumulation of mucins (neutral mucopolysaccharides dyed pink), we stained the sections with Periodic Acid-Schiff (PAS) and performed a hematoxylin counterstain. Slide observations were made using an optical microscope at × 200 magnification (BX51, Olympus).
The 4 μm thick sections, fixed in 10% formalin and subsequently embedded in paraffin, underwent deparaffinization and rehydration through an ethanol gradient. Antigen retrieval was performed using a microwave to treat the sections to Tris-EDTA Buffer (10 mM Tris Base, 1 mM EDTA Solution, 0.05% Tween 20, pH 9.0). Endogenous peroxidase activity was neutralized with 3% hydrogen peroxide, followed by a 30-minute blocking step using 2.5% normal horse serum. The following primary antibodies were used: 8-hydroxy-2'-deoxyguanosine (8-OHdG, Abcam, Waltham, MA, USA) and AGEs (TransGenic, Fukuoka, Japan). Primary antibody signals were detected using the VECTASTAIN Elite ABC Universal Kit (Vector Laboratories, Burlingame, CA, USA), and visualization was achieved with the DAB peroxidase substrate kit (Vector Laboratories). Counterstaining was performed with hematoxylin, and the samples were mounted. Tissue sections were incubated with nonimmune animal serum instead of the primary antibodies as a negative control. Observations were made using an optical microscope at 200 × magnification (BX51, Olympus). Quantification was done using ImageJ software to measure the average optical density (mm2) per unit area in five randomly selected areas at 200 × magnification (NIH).
The DPP4 activity and GLP-1 level were measured using the mouse DPP4 (MyBioSource, San Diego, CA, USA) and GLP-1 ELISA Kit (MyBioSource), following the manufacturer’s instructions. The results were measured using the Spark® Multimode Microplate Reader (Tecan, Männedorf, Switzerland).
RESULTS
Body weight was higher in the db/db mice at the endpoint, and blood glucose levels also increased in the db/db mice (Fig. 1A and B). Additionally, salivation decreased significantly in the db/db mice (Fig. 1C). The weight of the salivary gland reduced considerably in the db/db mice (Fig. 1D). H&E staining was performed to observe morphological changes in the salivary gland. The ductal area to serous acini area ratio increased in the salivary gland. In contrast, the location of serous acinar cells decreased in the db/db mice (Fig. 2A and B).
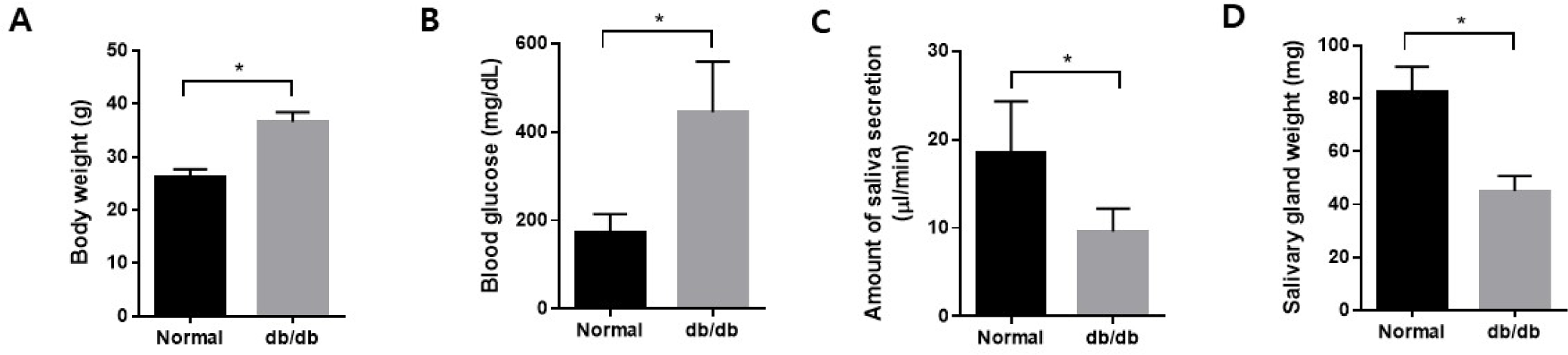
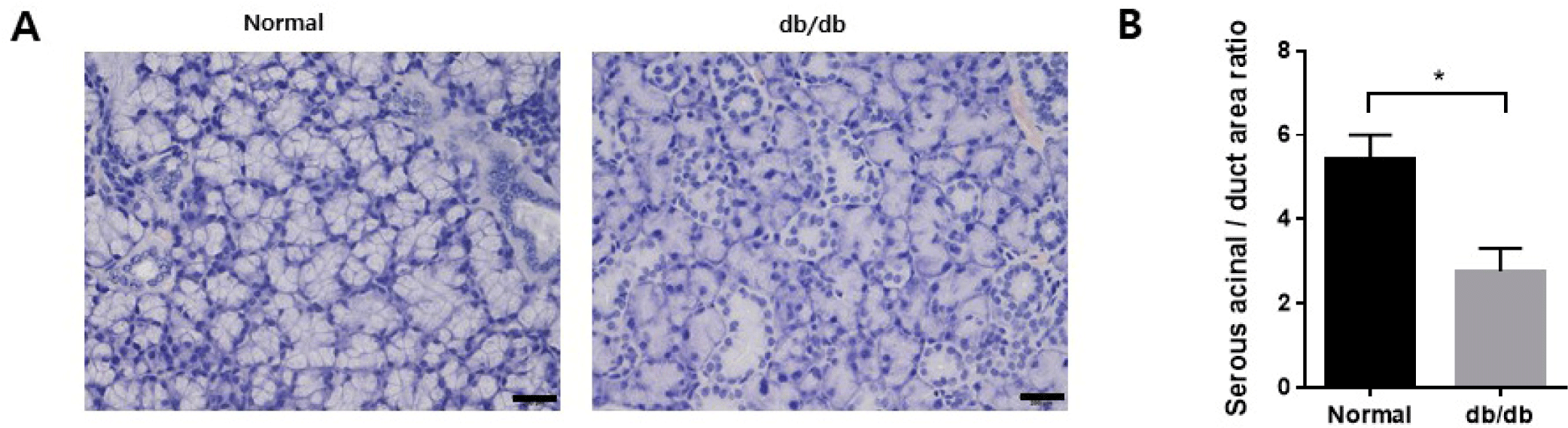
There was a significant increase in both salivary gland and serum DPP-4 activity in db/db mice compared to the normal mice (Fig. 3A and B). In contrast, GLP-1 levels in salivary glands and serum significantly decreased in the db/db mice compared to the normal mice (Fig. 3C and D). This could potentially have implications for DPP-4 and GLP-1 in salivary gland dysfunction.
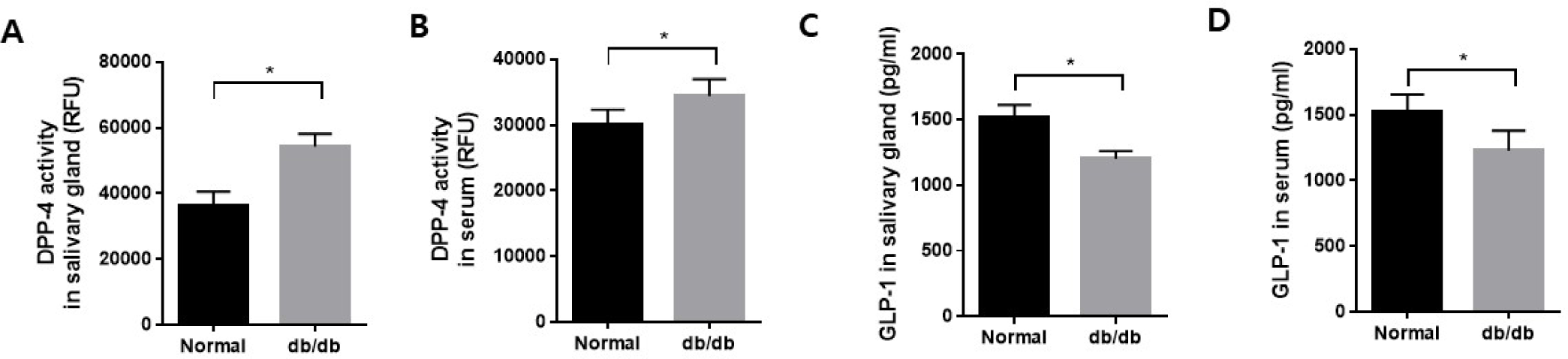
TUNEL assay was conducted to confirm apoptosis in the salivary glands. TUNEL-positive apoptotic cells were significantly increased in the db/db mice (Fig. 4A and B). Immunohistochemistry was conducted to assess the extent of 8-OHdG accumulation as a marker of cellular damage induced by oxidative stress. 8-OHdG presence was confirmed in the salivary glands. Accumulation of 8-OHdG increased in the db/db mice (Fig. 4C and D).
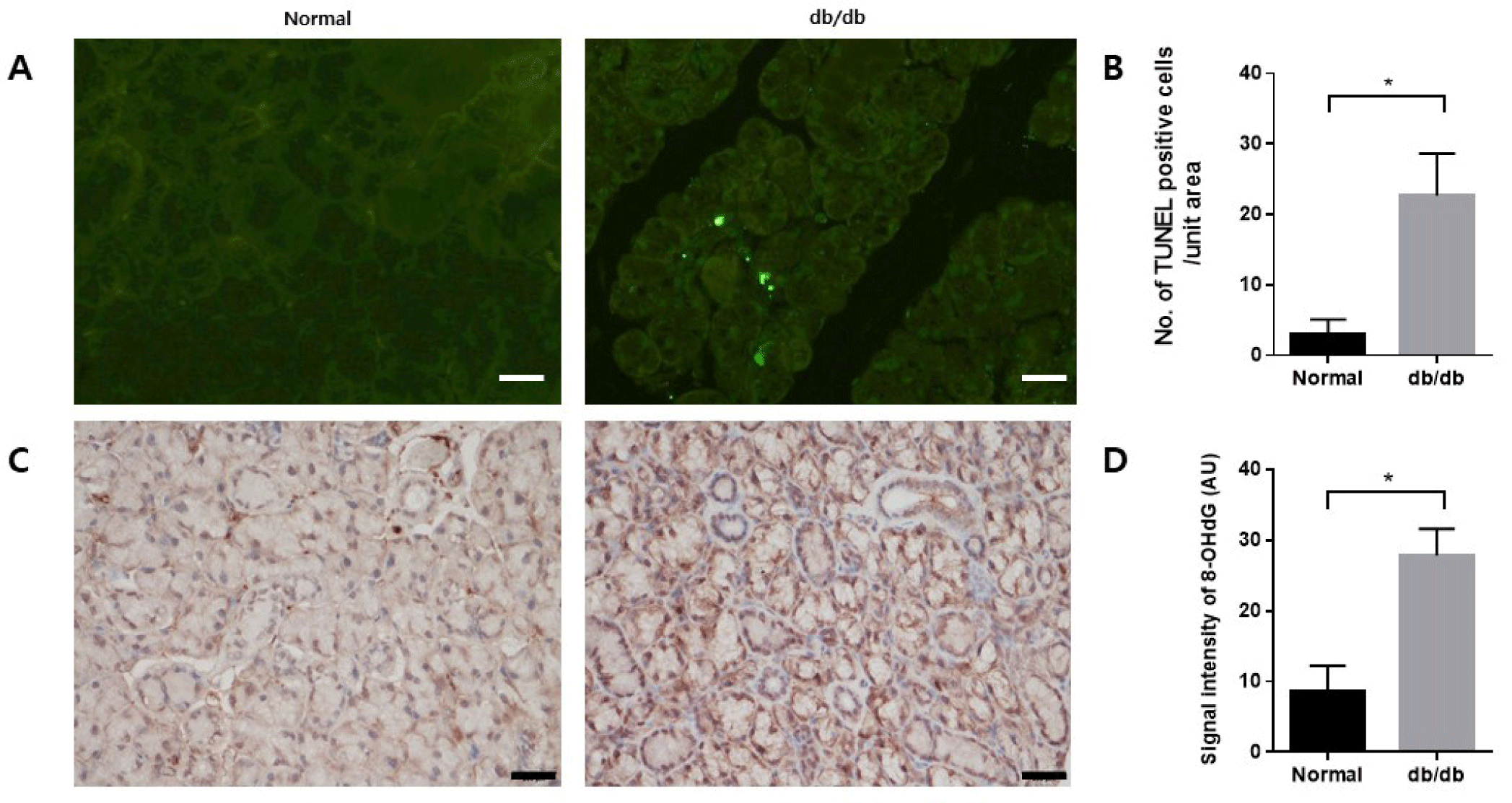
The accumulation of AGEs in the salivary glands was examined using immunohistochemistry. There was an increased accumulation of AGEs in the db/db mice (Fig. 5A and B). AGE levels were measured in salivary gland lysate and serum. The levels of AGEs increased in both salivary glands and serum in the db/db mice (Fig. 5C and D).
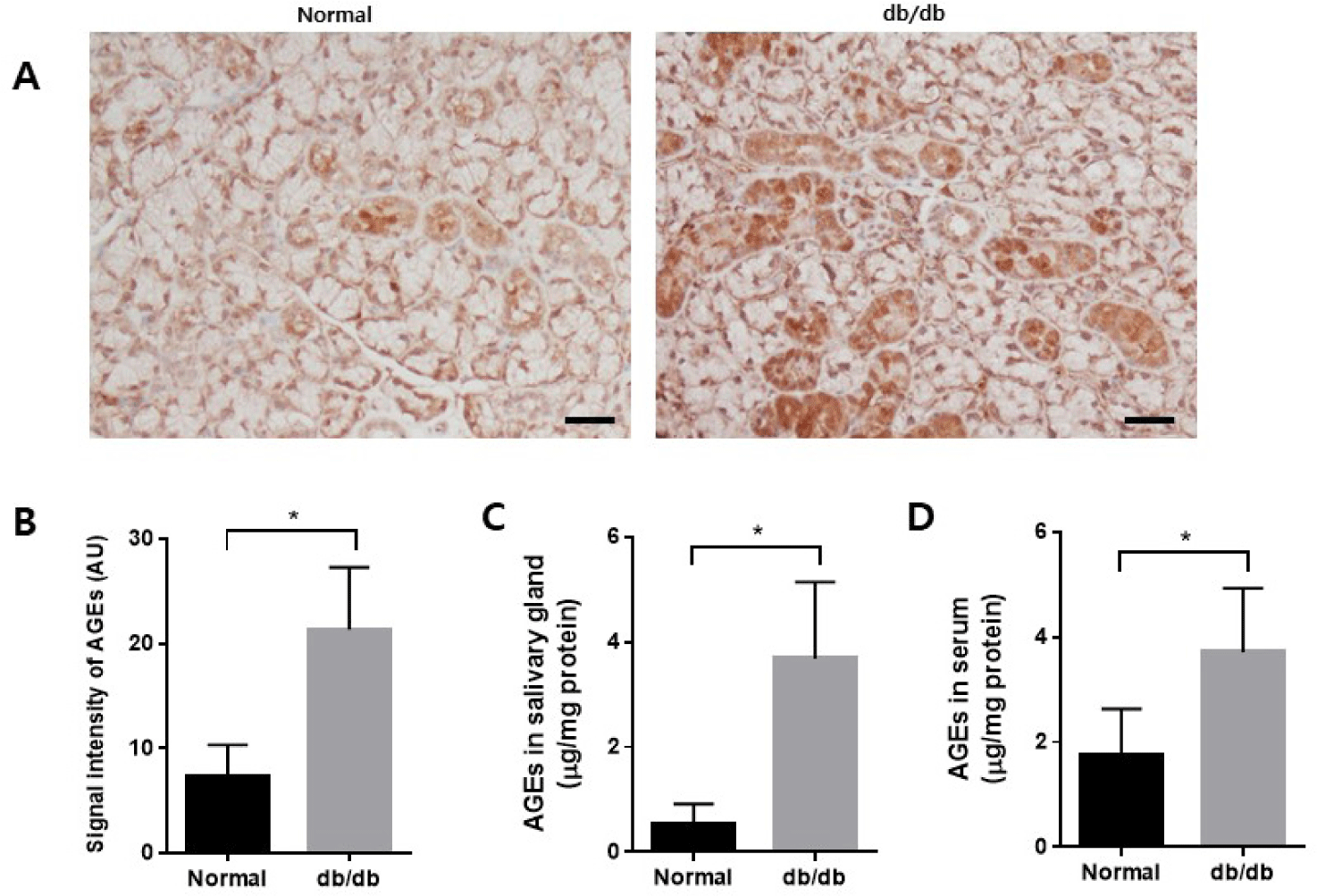
PAS staining was performed to confirm changes in mucin accumulation in salivary gland acinar cells. In the normal group, the acini showed moderate staining with PAS reagents. However, in the db/db mice, there was a noticeable increase in staining intensity, indicating a higher accumulation of mucin within the acini (Fig. 6A and B).
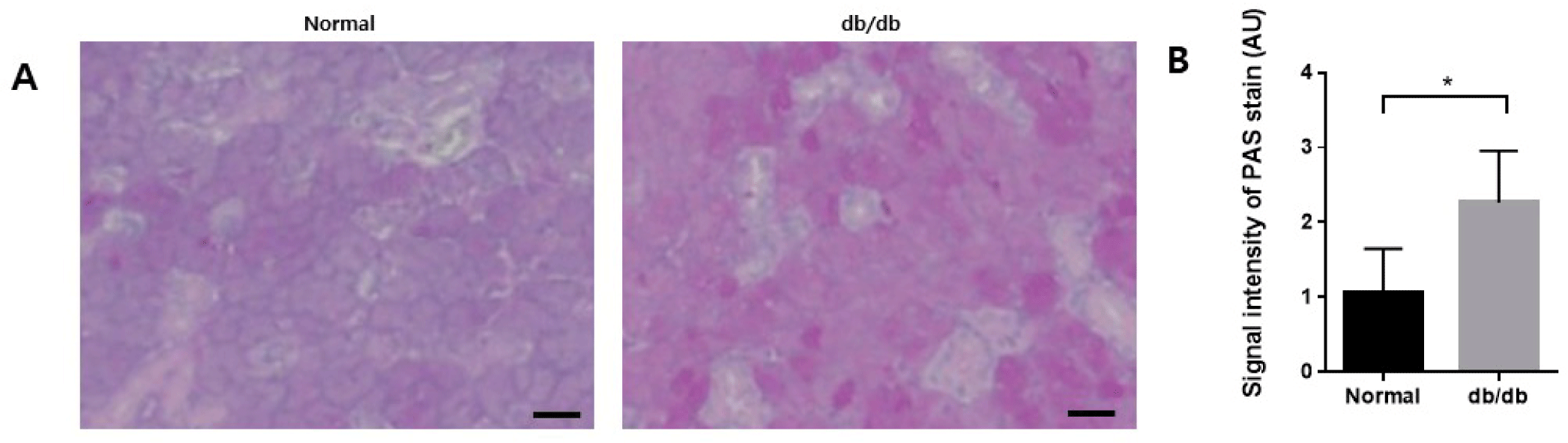
DISCUSSION
Saliva is essential in maintaining oral health homeostasis [1-3]. Without adequate saliva production, oral tissues become damaged and vulnerable to infection, which can lead to oral diseases [10]. The generation of reactive oxygen species (ROS) in the salivary glands results in acinar cell loss and decreased saliva secretion [9]. In this study, we investigated the role of DPP-4 and GLP-1 on salivary gland function in db/db diabetic mice.
DPP-4, also known as adenosine deaminase complexing protein 2 or CD26, degrades incretin hormones such as GLP-1 [22, 23]. DPP-4 inhibition improves metabolic control by enhancing GLP-1-mediated pancreatic insulin secretion and suppressing hepatic glucose production [24]. GLP-1 has a very short half-life and is rapidly metabolized by DPP-4 [25-27]. GLP-1 is an incretin hormone secreted from the gut lumen that enhances insulin secretion [28-30]. Increasing endogenous levels of GLP-1 may improve glycemic control in individuals with type 2 diabetes and could potentially serve as a treatment for diabetes-related complications. We first confirmed increased DPP-4 activity and decreased GLP-1 levels in the salivary glands and serum of db/db mice, demonstrating their function within the glands.
Studies have shown that hyperglycemia can increase intracellular ROS through glycation reactions of amino acids or proteins, glutathione depletion, and inactivation of ROS scavenger enzymes. This can result in mitochondrial dysfunction and apoptosis [31-35]. Hyperglycemia-induced oxidative stress can be blocked by antioxidants, suggesting that ROS plays a vital role in hyperglycemia-induced cell death [36, 37]. We showed apoptosis in salivary glands with elevated levels of ROS. Increased ROS may be caused by increased DPP-4 and a decrease in GLP-1. GLP-1 plays a role in glucose-stimulated insulin secretion and gluconeogenesis, improving glycemic control in individuals with type 2 diabetes [24]. GLP-1, acting as an antioxidant, can suppress ROS production and inflammation in endothelial cells during hyperglycemia and in animal models of diabetes [38, 39]. DPP-4 increases ROS production in endothelial cells, and DPP-4 deficiency inhibits ROS production in cardiomyocytes [40, 41]. DPP-4 inhibitors reduce ROS production, prevent mitochondrial dysfunction, improve endothelial function, and reduce vascular inflammation and oxidative stress in animal models of diabetes and its complications [42-45]. A DPP-4 inhibitor, gemigliptin, has been reported to improve liver disease and diabetes by alleviating mitochondrial dysfunction and reducing ROS production [46, 47]. Based on previous studies and our results, DPP-4 and GLP-1 are attributed to the increase of ROS.
In addition, there was increased accumulation of AGEs in the salivary glands of db/db mice. In a previously reported study, AGEs formation inhibition by increased GLP-1 in DPP-4-deficient mice improved diabetes and diabetic complications [48, 49]. Additionally, numerous existing studies have verified the antiglycation effect of many DPP-4 inhibitors [17-20, 50]. Further, gemigliptin prevented a decline in salivary gland function through its antioxidant effect in diabetic rats [21]. We observed increased DPP4 activity, decreased GLP-1 levels, and elevated AGE accumulation in db/db mice. These factors subsequently induced ROS and, ultimately, apoptosis in salivary gland cells.
We observed morphological differences in the ratio of acinar cells to ductal cells in the salivary glands. Db/db mice showed decreased acinar cells and increased ductal cells in their salivary glands relative to the control group. Similar results have been observed in diverse salivary gland dysfunction models. The diabetes-related reduction in secretory cells in salivary glands is thought to result from decreased cell proliferation or increased cell death [38]. We measured salivary flow after administering pilocarpine, a parasympathetic agonist on muscarinic acetylcholine receptors in salivary glands [42]. The increase in ROS-induced apoptosis caused by diabetes may lead to salivary gland dysfunction. Oxidative stress, caused by ROS accumulation, altered secretion functions, such as saliva secretion, salivary amylase, and calcium levels, in rodent salivary glands [45]. These findings suggest that ROS-related oxidative stress can lead to salivary secretion disorders.
In our study, strong PAS staining demonstrated increased mucin accumulation in the acini in the db/db mice. Mucin plays a crucial role in mucosal lubrication, serving as one of the primary functions of saliva. Mucin can hold significant water, preventing mucosal dehydration [51, 52]. Mucins are vital components of saliva, lubricating and facilitating microbial adhesion. Mucus accommodates microbial communities and forms a protective coating on epithelial surfaces, playing a significant role in host defense [53]. Our results showed that mucin accumulated in acini with salivary gland dysfunction. Previous reports have shown that stronger PAS staining density in acini is associated with the accumulation of mucin in diabetic salivary glands [54-56]. Mucin composition in salivary glands can change due to salivary gland dysfunction [57]. Huang et al. also showed that acini of submandibular glands were moderately stained by PAS reagents in db/m mice but strong staining was observed in db/db mice. Stronger PAS staining densities of acini in db/db mice demonstrated that more mucins accumulated in the acini of diabetic salivary glands [54]. Therefore, mucus accumulation under diabetic conditions provides evidence that the secretory function of the salivary glands is impaired .
The limitation of this study is that we only showed the impaired DPP-4 activiry and GLP-1 levels under diabetic conditions. To better understand diabetes-related salivary dysfunction, additional investigation is required to elucidate the effect of GLP-1 recovery by DPP-4 inhibition using a selective DPP-4 inhibitor.
In conclusion, we have shown the possible involvement of DPP-4 and GLP-1 in increased ROS-induced apoptosis in diabetes-related salivary gland dysfunction. The pathological changes induced by DPP-4 and GLP-1 in salivary glands decrease the amount salivary secretion and increase the accumulation of mucins in the salivary glands due to decreased secretory. DPP-4 and GLP-1 could be a potent pharmacological target for patients with diabetes-related salivary gland dysfunction.