INTRODUCTION
Globally, renal stone disease is a growing and alarming problem. Changing dietary habits and lifestyles have resulted in an increase in the frequency of this disease [1]. The lifetime risk for nephrolithiasis exceeds 6% to 12% in the general population [2], and it is also projected that the prevalence of kidney stones will escalate. Nephrolithiasis may be associated with an increased risk of end-stage renal disease [3], coronary artery disease, the metabolic syndrome, hypertension, and diabetes mellitus [4]. Hyperoxaluria, an excessive urinary oxalate excretion, has been linked to this disease [5]. Deficits of the hepatic peroxisomal enzyme alanine glyoxylate aminotransferase cause hyperoxaluria, a disease in which oxalate is constantly overproduced, causing recurrent kidney stones, progressive nephrolithiasis, renal failure, and elevations of plasma oxalate, which in turn leads to calcium oxalate (CaOx) crystals accumulating in the urinary system. There is a high prevalence of urinary calculi caused by CaOx around the world [6]. Increasing urinary supersaturation and the formation of solid crystal particles are responsible for forming CaOx in the urinary tract. [7]. To treat kidney stones, lithotripsy and surgical methods were applied [8]. There are some inhibitors of kidney stone formation. Some of the known inhibitors are alkaline pH, citrate, pyrophosphate, magnesium and glycoproteins such as osteopontin, Tamm-Horsfall protein, urinary prothrombin fragment 1, glycosaminoglycans, and nephrocalcin [9].
Normally, oxalate is eliminated from the body through the kidneys [10]. Under conditions of renal insufficiency, the gastrointestinal tract provides an alternative route for oxalate elimination [11]. Hoppe et al. showed that Oxalobacter formigenes, a microflora found in the intestinal tract, may limit oxalate absorption or promote its excretion from the colon [12]. This result suggests that pharmacological manipulation of oxalate in the intestinal tract may affect hyperoxaluria and renal stone disease. However, there are risks associated with the use of this bacteria for renal stone disease. O. formigenes is an anaerobic bacterium. Factors affecting viability during storage such as temperature, moisture, and air should also be taken into consideration. Oxygen is a major problem in the survival of anaerobic bacteria [13]. Probiotic bacteria can interact with commensal bacteria and can also have a direct impact on the host. These risks are mainly concerned with respect to safety in vulnerable target groups such as immune compromised individuals (pregnant women, babies and the elderly) [14]. There are few effective therapeutic options for hyperoxaluria. In the present study, we examined whether a synthetic oxalate-degrading enzyme, oxalate decarboxylase (OxdC) inhibits the formation of CaOx crystallization in vitro and prevents CaOx nephrolithiasis induced by ethylene glycol (EG) in rats.
MATERIALS AND METHODS
Crystallization of CaOx was induced by adding 4 mM calcium chloride and 50 mM sodium oxalate to artificial urine (0.05 mM Tris and 0.15 mM NaCl, pH 6.5) for 1 hour at 37℃. The crystallization of CaOx was determined by optical density at 620 nm with or without OxdC (Genofocus, Daejeon, Korea). The percentage inhibition was calculated as experimental optical density/control optical density/100. To identify the size and shape, the crystallization of CaOx was also observed under an optical microscope (BX51, Olympus, Tokyo, Japan).
Male Sprague-Dawley rats aged 6 weeks were purchased from Koatech (Seoul, Korea). Rats were housed under a 12-h light/dark cycle at a temperature of 23 ± 1℃ and 55 ± 5% humidity and were provided with standard rodent pellets and filtered water ad libitum. The rats were then randomly divided into 3 groups as follows: normal control group (CON, n = 7), EG-induced nephrolithiasis group (EG, n = 7), and OxdC-treated group (OxdC, n = 7). The rats in the EG group were orally administered with 6 g/kg/day EG (Sigma-Aldrich, St. Louis, Mo, USA). The rats in the OxdC group were orally administered with 6 g/kg/day EG and 10 units/kg/day OxdC at the same time. The rats in the CON group were administered with distilled water. The dose of EG (6 g/kg/day) was also determined according to several previous studies’ protocols [15, 16]. In the previous, study, OxdC (1,000 units/day) were administered in human [17]. Based on the clinical human's dose of OxdC, the rat equivalent dose was approximately equal to 80 units/kg. However, in the present study, a lower oral dose (10 units/kg/day) was determined considering safety. All animals were sacrificed 3 weeks later. In order to conduct the animal study, the Institutional Animal Care and Use Committee of Jeonbuk National University Hospital Laboratory Animal Center approved the protocol (JBNUH 2024-005).
Urine samples were collected in metabolic cages 24 hours before the sacrifice. Blood serum samples were collected at the sacrifice. Serum creatinine was measured with a creatinine assay kit (Abcam, Waltham, MA, USA). Serum urea nitrogen was measured with a urea nitrogen detection kit (Thermo Fisher Scientific, Waltham, MA, USA). Urinary oxalate was measured with an oxalate assay kit (Sigma-Aldrich).
The histology evaluation was conducted with Hematoxylin and Eosin (H&E) using the kidney sections. A microscope was used for observation of the slide (BX51, Olympus). An examination and scoring of renal sections were conducted in order to assess the degree of renal damage. We evaluated histological damage using the endothelial, glomerular, tubular, and interstitial (EGTI) scoring method, which was specifically developed for histological analysis of kidney tissue after injury [18].
Kidney sections were also stained by lead nitrate and ammonium polysulphide method, which is a standard procedure to stain CaOx [19]. To define the severity of nephrolithiasis, scoring was done as follows: 0 = no oxalate crystals in any area; 1 = 1–9 crystals in any field, and 2 = all fields with multiple depositions of crystals.
Following Levene's test, a one-way ANOVA was performed, and significant differences between groups were detected. According to the homogeneity of dispersion, a post-hoc test was conducted (Tukey's multiple comparison test for homogeneous dispersion or Dunnett's T3 test for heterogeneous dispersion) using a Graphpad Prism 8.0 software (GraphPad, San Diego, CA, USA). We expressed all data as means ± S.E. A p-value less than 0.05 was considered significant.
RESULTS
The in vitro inhibitory effect of OxdC on CaOx crystallization was determined by the optical density of turbidity. OxdC at various concentrations had a significant inhibitory effect on the crystallization of CaOx. There was a concentration-dependent decrease in the absorbance with increasing concentration of OxdC (Fig. 1A). Light microscopy showed the formation of both types of CaOx crystals with oval round edges or sharply jagged edges in the artificial urine (Fig. 1B). OxdC at the highest concentration (100 mU/mL) inhibited crystal formation as well as a few crystals with sharply jagged edges.
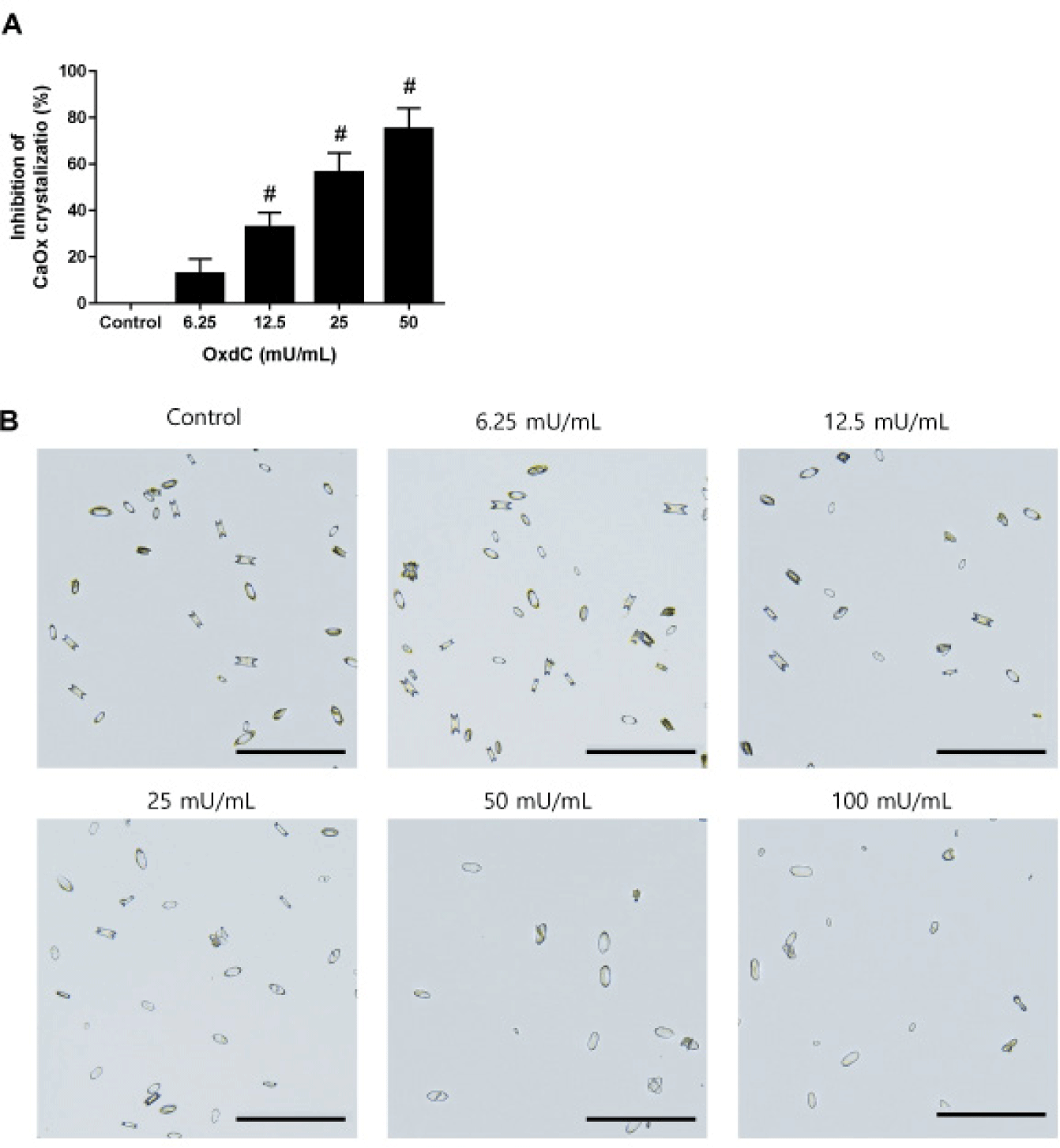
Serum urea nitrogen and creatinine have typically been used to evaluate renal function. Fig. 2 showed that serum urea nitrogen and creatinine were significantly higher in the EG group than in the CON group. However, OxdC treatment significantly lowered serum urea nitrogen and creatinine levels compared with the EG group. The oxalate assay revealed significantly higher levels of urinary oxalate in the EG group than in the CON group. However, oral administration of OxdC resulted in significantly decreased levels of oxalate in urine compared with the EG group (Fig. 3).
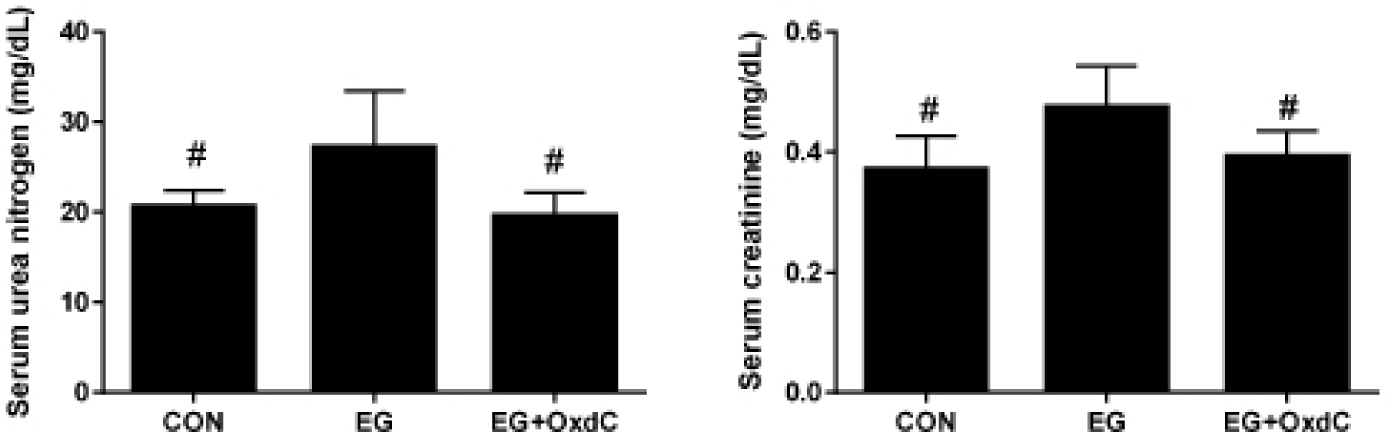
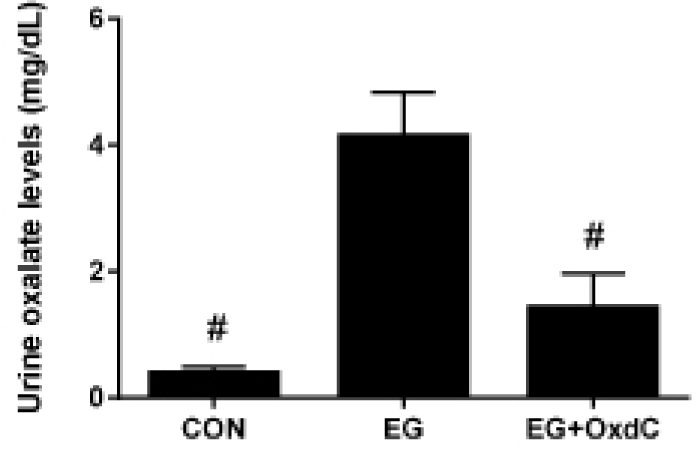
As shown in H&E staining, both the renal cortex and medulla of EG-treated rats had distorted glomeruli with damaged and extremely dilated tubules. There was no abnormal microstructure in the renal cortex or medulla of the rats in the CON group. All these damage signs and EGTI scores were improved in the OxdC group (Fig. 4). Histological evaluation with CaOx staining demonstrated that normal kidneys in the CON group had no evidence of nephrolithiasis. However, the rats in EG had an increase in CaOx deposits in the cortex and medulla. The severity score of nephrolithiasis was significantly decreased in the OxdC group (Fig. 5).
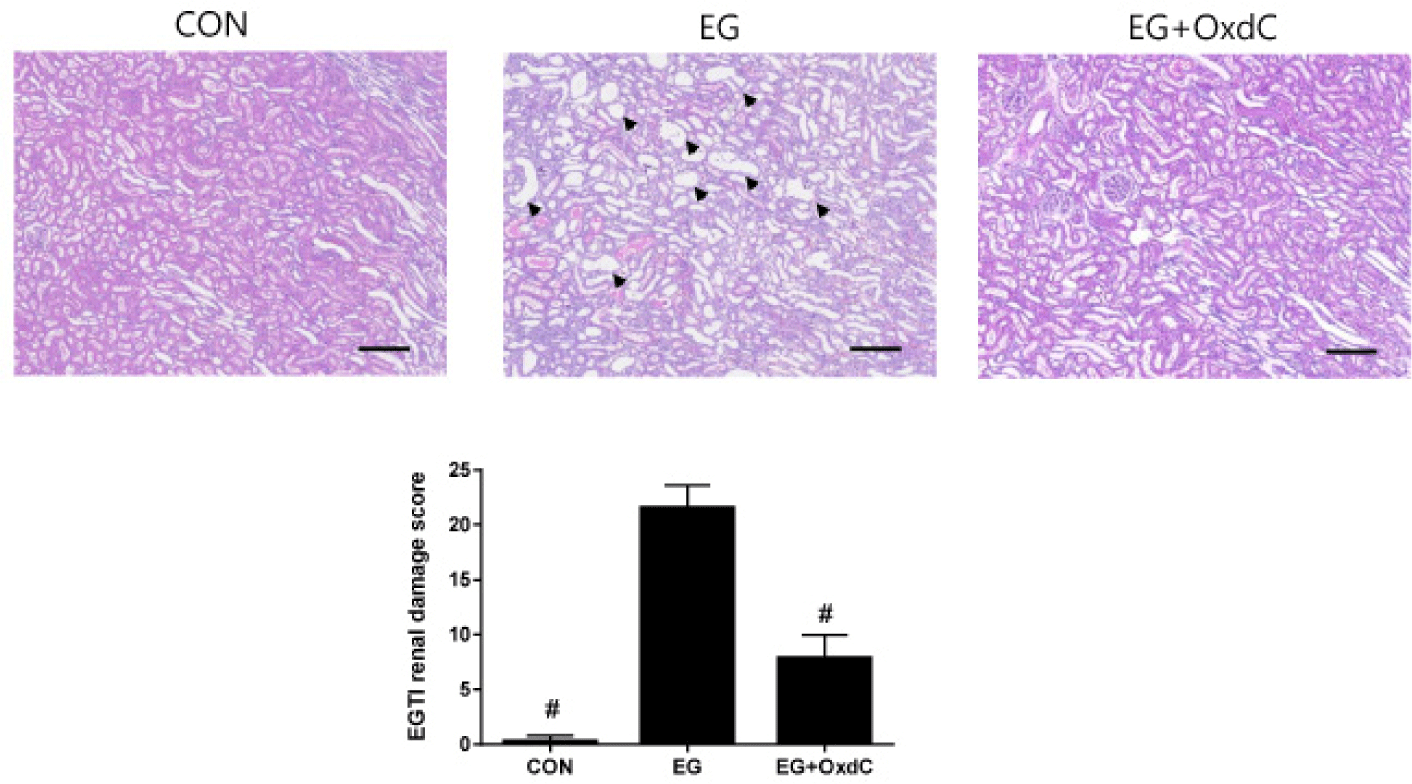
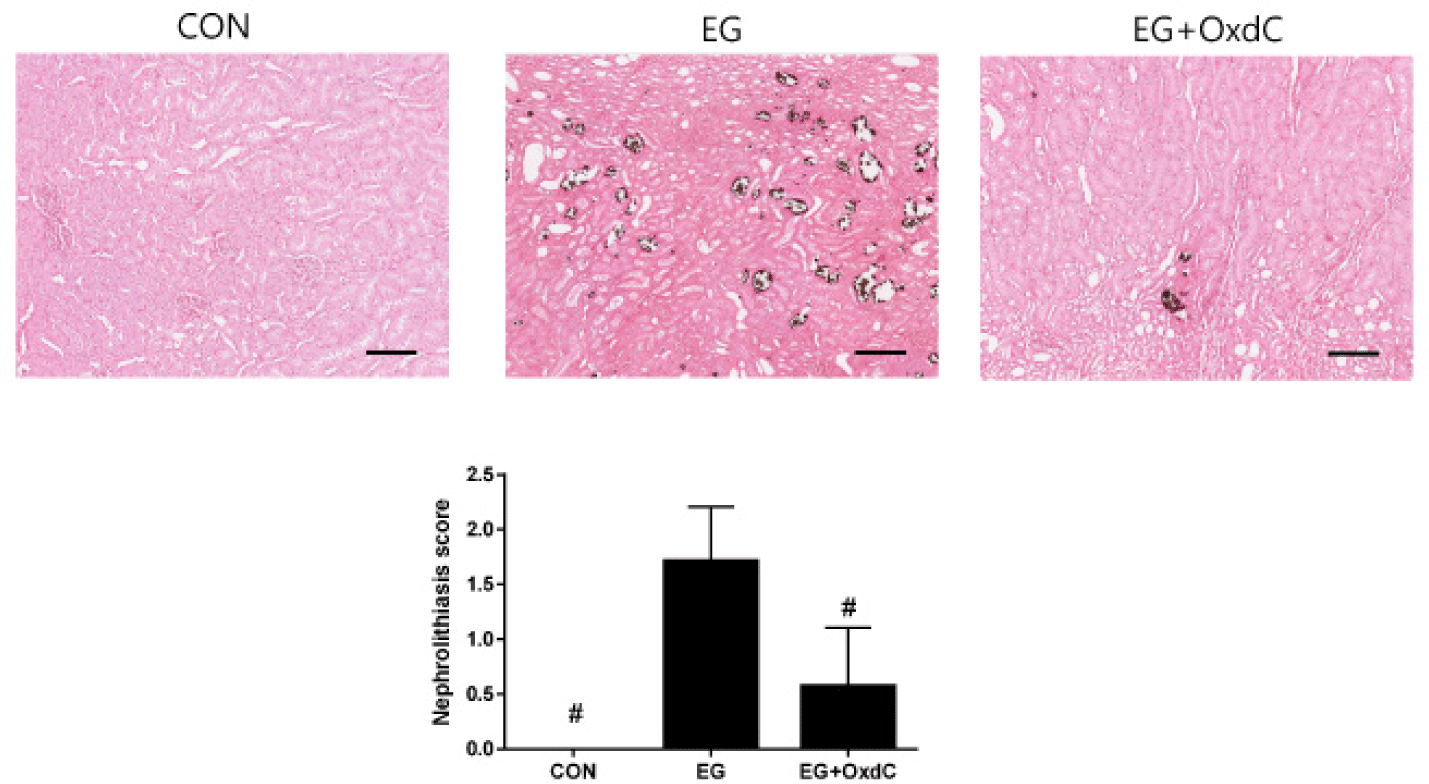
DISCUSSION
It is estimated that approximately 12 percent of the world's population suffers from kidney stones disease [20]. There has been extensive research into the fundamental mechanisms underlying renal stones, which reveals that there is an imbalance between stone inhibitors within the urine and the crystallization process. [21]. Since kidney stones are very prevalent and have negative consequences for patients, there is a growing movement for proper management.
There are some inhibitors of kidney stone disease. However, the current pharmacological options have several limitations, such as lack of efficacy, stone recurrence, possible increased risk of allergic reactions of diuretics [2]. It is important, however, to explore new pharmacological options to ensure safe and effective management [22].
Based on a classical model of calcium chloride and sodium oxalate supersaturated synthetic urine, CaOx crystallization was studied. When OxdC was treated in vitro, the formation of CaOx crystals was inhibited in a concentration-dependent manner. Moreover, OxdC induced the formation of a few crystals with sharply jagged edges. Among individuals, there are often differences in the shape and size of kidney stones due to the supersaturation of lithogenic salts, pH value, and inhibitors and promoters present in their urine [23, 24]. Crystal sharpness should also be an important factor that influences cellular injury when crystals are exposed to cells. It is easy for cell membranes to rupture when crystals have sharp edges. Cell injury was aggravated by sharp crystal aggregation, while that was weakened by blunt crystal aggregation [24]. Our in vitro assay clearly showed that Oxdc may have a potent inhibitory effect on renal cell injury by inhibiting CaOx crystallization as well as inducing the formation of blunt crystals at the same time.
In the animal experiment, we used a rat model for EG-induced CaOx nephrolithiasis. There are many poisonings linked to EG, which is found in antifreeze [25]. Acute renal failure is caused by its transformation into oxalic acid by the liver. Oxalic acid precipitates in the kidneys as CaOx, resulting in its toxicity. We evaluated whether enzymatic blocking of oxalate by OxdC could be a pharmacological option for hyperoxaluria and nephrolithiasis in this animal model. In the current study, EG treatment resulted in hyperoxaluria and kidney function impairment, based on high serum urea nitrogen and creatinine levels. The results indicate that EG-treated rats had impaired renal and glomerular filtration. Additionally, a significant development of distal convoluted tubule damage and dilation, along with increased deposition of CaOx crystals and stones, was also observed in rats that received EG treatment. This nephrolithiasis characterized by CaOx intratubular crystal deposits with hyperoxaluria in EG-treated rats was dramatically decreased by oral administration of OxdC. It also shows that maintaining kidney function is linked to reducing hyperoxaluria and preventing nephrolithiasis. By reducing urinary oxalate levels with OxdC, rats showed decreased CaOx crystal deposition in the kidney, as well as maintaining kidney filtration function. This study had some potential limitations. First, single dose of OxdC was examined in the animal study. Second, the in vivo stability of OxdC in the intestinal tract is unknown. Thus, we have a future study plan that can clearly identify the effective oral dose of OxdC and its intestinal stability.
In conclusion, the present study provides evidence that oral treatment with the oxalate-degrading enzyme, OxdC, significantly inhibited CaOx crystallization and improved hyperoxaluria as well as CaOx nephrolithiasis. We believe that oral administration of OxdC is a potential therapy in the treatment of human hyperoxaluria as well as CaOx nephrolithiasis.