INTRODUCTION
Corticosterone (CORT), a steroid hormone produced in the adrenal cortex, is the main glucocorticoid found in many vertebrates, including species of rodents, birds, and reptiles, and cortisol is the primary glucocorticoid in humans. CORT is induced in mice by acute and chronic stress, and both types of stress increase the plasma concentration of CORT [1]. CORT has many physiological functions involving energy metabolism, immune reactions [2], and even memory [3]. It is also related to plasma lipids, especially cholesterol, in rats. Stress such as lever press escape/avoidance induced the increase and decrease in plasma and aortic cholesterol levels, respectively, while accompanying the elevation of CORT [4].
Stress can be mainly classified into two types, acute and chronic, which cause different physiological changes. For example, plasma metabolites and the responses to chronic stress were dependent on model species and stress-induction (SI) methods [5]. Even acute stress is also controversial subject, which the results were influenced by the species of target animal, stress-duration time, stressor type, and other experimental conditions [6]. Moreover, with regard to lipid distribution and metabolism, acute and long-term effects of glucocorticoids within tissues and tested models remain unclear and controversial [7].
Oxidative stress is defined as an imbalance between various types of free radicals, such as reactive oxygen species (ROS) and antioxidants in cells, leading to damages in proteins, lipids, cell membranes, and DNA. Therefore, it affects the pathogenesis of a wide range of diseases, particularly including diabetes, neurological disorders, and cancers [8]. Oxidative stress is generated from endogenous immune activation, inflammation, infection, exercise, aging, and stress, as well as exogenous drugs, smoking, alcohol, pollutants, and radiation [9]. Many studies have demonstrated that some of cytochrome P450 (CYP) enzymes, such as CYP1A, CYP3A, and CYP2E1, play an important role in the production of oxidative stress during drug metabolic reactions in animals [10].
Korean Red Ginseng (KRG, Panax ginseng Meyer) is one of the important traditional medicines. Various studies reported the effects of ginseng compounds on physiological functions, including immunity, circulation, inflammation, and various other diseases [11]. KRG is also a well-known antioxidant, reducing ROS generation and increasing antioxidant enzymatic activities such as catalase and glutathione peroxidase [12]. Accordingly, KRG has been suggested to alleviate oxidative damages of organs and tissues such as the liver and the kidney and diverse diseases, which are closely related to oxidative stress [13]. Placebo-controlled study on human chronic stress suggested that KRG stabilizes the sympathetic nervous system and improves cognition in highly stressed individuals [14].
In this study, we investigated the stress-induced changes in the lipid and hormonal concentrations in mouse plasma and CYP-derived oxidative stress, as well as the enzyme activities in the liver. In addition, the anti-stress effects of KRG water extract were also assessed in relation to CYP activities, oxidative stress, and inflammation.
MATERIALS AND METHODS
KRG (Korea Ginseng Corporation, Daejeon, Korea) water extract was prepared using the roots of Panax ginseng, as described previously [15], which contained compounds such as Rb1 (7.44 mg/g), Rb2 (2.50 mg/g), Rc (3.04 mg/g), Rd (0.91 mg/g), Re (1.86 mg/g), Rf (1.24 mg/g), Rg1 (1.76 mg/g), Rg2s (1.24 mg/g), Rg3r (1.39 mg/g), Rg3s (2.41 mg/g), and Rh1 (1.01 mg/g).
Male C57BL/6 mice were obtained from Samtako Bio Korea (Osan, Korea). Mice (8 weeks old, 18–20 g) were divided into 5 groups: normal (unstressed, control), SI, and SI-KRG: SI-KRG5, SI-KRG20, and SI-KRG50 indicated SI groups co-treated with 5, 20, and 50 mg/kg/day of KRG extract, respectively. All mice were used after one week of quarantine and acclimation. Seven mice were housed per cage in a temperature-controlled room (23 ± 1°C) and lighting (alternating 12-hour light and dark periods). Mice were fed a restricted calorie diet (approximately 13 kcal per mouse per day) during all experimental periods by providing Laboratory Rodent Diet 5001 (LabDiet, St. Louis, MO, USA) due to a close relation between plasma levels of lipid including hormones and calorie intake. The restricted calorie was ensured using the mice that consumed the allocated diet completely. The experimental design was approved by the committee for the Care and Use of Laboratory Animals at Chonnam National University (CNU IACUC-YB-2021-142).
Stress was induced in mice using a restraint method as described [16] with a modification. Briefly, mice were introduced into a small custom-made acryl cylinder (restrainer) with open head and tail gates. The mice were then almost immobilized in the restrainer for 120 min. During the recovery periods after stress induction, mice were allowed ad libitum access to water and the diet under normal housing conditions. The stress induction was subjected to each group of 7 mice three times, once a day for 3 days. Unstressed mice were used as controls. KRG was orally fed to mice once a day for 8 days, including pre-stress and the SI periods. Since the oral administration possibly affected the observed results, the same amount of water with the KRG extract was orally fed to mice in the control and SI groups.
Twelve hours after final stress induction and KRG treatment, mice were anaesthetized and sacrificed using sodium pentobarbital (50 mg/kg) injection. Blood was taken from the inferior vena cava and collected into two tubes, one of which contained potassium EDTA to measure the plasma concentrations of triglycerides (TG), total cholesterol (TC), and lipoprotein cholesterol, such as high-density lipoprotein-cholesterol (HDL-C) and low-density lipoprotein-cholesterol (LDL-C). The other tubes were heparinized to analyze the plasma concentrations of CORT, insulin, and glucagon. Blood plasma was obtained by centrifugation at 4°C. Glucose concentrations were measured using a glucose oxidase reagent set (Pointe Scientific, Canton, MI, USA). TC level was measured by a Pureauto S assay kit (JW Pharmaceutical, Seoul, Korea). HDL-C and TG levels were measured using ADVIA 2400 (Siemens, Washington, DC, USA). LDL-C concentration was calculated by the modified Friedewald equation [17]. Plasma CORT was analyzed by high-performance liquid chromatography-fluorimetry [18]. Insulin concentration was measured using ADVIA CentaurTM Insulin Lite Reagent and Solid Phase (Siemens), and detected by ADVIA CentaurTM XP (Siemens). CORT concentrations were assayed by the VetTest chemistry analyzer (IDEXX, Westbrook, ME, USA). The C-reactive protein (CRP) concentrations were analyzed using dedicated ELISA kits (Abcam, Cambridge, UK). Total ROS levels in the plasma and cytosolic fractions of the liver were measured using a fluorometric intracellular ROS kit (Sigma-Aldrich, St. Louis, MO, USA).
Cytosolic and microsomal fractions of liver cells were obtained using a previously described method [19]: immediately after decapitation, the liver was excised, rinsed in liquid nitrogen, diced, and homogenized in 2–5 volumes of 100 mM Tris-HCl (pH 7.4, 4°C) containing 2 mM phenylmethyl sulfonyl fluoride and pepstatin (12.5 μg/mL), a protease inhibitor, using a homogenizer (IKA, Staufen, Germany). The homogenate was centrifuged at 10,000 × g for 20 min (4°C), and the resultant supernatant was centrifuged at 200,000 × g for 60 min (4°C) to sediment the microsomes. The supernatant was used as the cytosolic fraction, and the microsomal pellet was suspended in 100 mM Tris-HCl (pH 7.4, 4°C).
CYP1A2 enzyme activity were measured using the P450-Glo CYP1A2 Assay (Promega, Madison, WI, USA). To measure the activity of CYP2E1, the p-nitrophenol hydroxylase activity was measured as reported [20]. CYP3A4 activity was measured using testosterone as described [21]. Microsomal fractions of liver were used for all CYP enzyme assays. Total protein concentrations were determined using Bradford assay (Sigma-Aldrich) with bovine serum albumin as the protein standard.
Total RNA was extracted from the liver extract using the RNeasy Mini Kit (Qiagen, Hilden, Germany). The extracted RNA was used to synthesize first-strand cDNA using the Reverse Transcription System (Promega) according to the manufacturer’s protocol. Quantitative real-time polymerase chain reaction (qRT-PCR) was performed using Rotor-Gene 6000 (Qiagen), and the 2−∆∆CT method was used for relative quantitation. Threshold cycle number and reaction efficiency were determined using Rotor-Gene 6000 series software version 2.7. The primers for CYP1A2, CYP2E1, CYP3A4, and glyceraldehyde 3-phosphate dehydrogenase (GAPDH) genes were obtained from OriGene (Rockville, MD, USA).
RESULTS
The clinical signs, such as mortality and body weight, were first examined as a preliminary test. Treatment-related mortality and body weight, which were statistically significant, were not observed in all mice tested during the study period (results not shown). Table 1 summarizes the plasma concentrations of hormones and lipids, including ROS and CRP, measured in the present study for all tested groups. When the control and SI group were compared, overall results paralleled the previous report [22]: the stress increased the plasma levels of CORT, TC, glucose, LDL-C, ROS, and CRP, whereas there were no noticeable changes in TG concentrations. The increased degrees for CORT and TC were 4.6- and 2.3-fold higher than the control, respectively. In particular, the total plasma ROS level in the SI group was remarkably enhanced by 5.4-fold compared to normal mice, suggesting that the stress elicited excess oxidative stress formation. As ROS are key signaling molecules that can induce inflammation progression [23], the concomitant CRP concentration was also elevated by 2.8-fold by the stress. Therefore, although other inflammation indices were not analyzed, these results imply the occurrence of a stress-induced pro-inflammation reaction, which has already been reported [24]. However, the enhanced glucose level was not significant, compared with other augmented parameters, which showed a 24% increase on average. On the contrary, the stress reduced the insulin and HDL-C levels by approximately 33% and 15%, respectively. When glucagon concentrations were measured, as it has an antagonistic relation with insulin, no discernible change in the parameter was observed as the result of the stress induction.
Psychological stress is associated with various pathological conditions, resulting in liver injury through multiple pathways, such as inflammation and oxidative stress [25]. Fig. 1A shows that the stress augmented ROS production in the liver extract by 4.3-fold, compared to the control. As the stress-prompted glucocorticoids are well recognized to produce ROS in the liver cells [26], this result demonstrated that the enhanced formation of ROS is closely related to the increased CORT. However, as total ROS was measured in this study, the types of ROS generated were not revealed. Furthermore, more detailed molecular mechanisms for CORT-mediated ROS production in the liver were not clear.
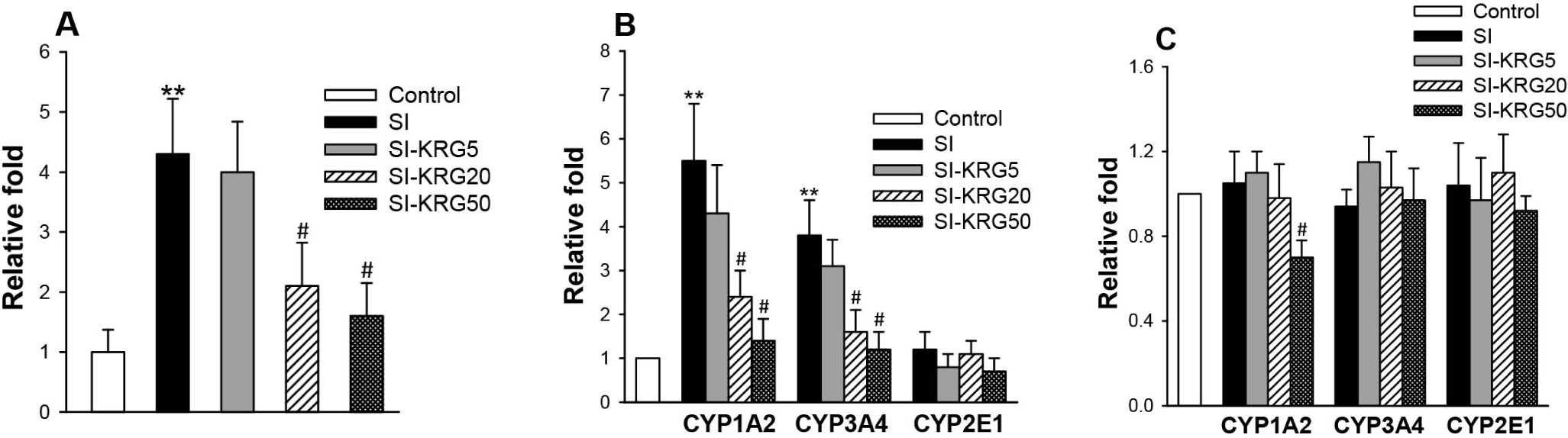
Fig. 1B shows that the stress stimulated the catalytic activities of CYP1A2 and CYP3A4 enzymes in microsomes by 5.5-fold and 3.8-fold, respectively, compared to the control. On the contrary, when the transcriptional level was measured by qRT-PCR analysis, stress did not promote a significant increase in CYP1A2 and CYP3A4 expressions, compared to normal mice (Fig. 1C). CYP1A and CYP3A enzymes have been known to generate significant ROS during drug metabolism [10] and CYP2E1 can also produce ROS, which is involved in the hepatocellular injury [27]. However, any noticeable changes in the CYP2E1 activity was not observed in SI group, compared to the control (Fig. 1B). Based on these results, it was anticipated that the stress-mediated stimulation of CYP1A and CYP3A4 activities might be attributed to the higher ROS production in the liver of SI group, although possible involvement of other CYP enzymes in the ROS generation could not be excluded [10].
Table 1 also indicates that KRG treatment to the SI group reduced the elevated CORT, TC, LDL-C, ROS, and CRP levels, which the alleviation was remarkable for ROS and CRP. However, the resultant values were still higher than those in normal mice. The diminished degrees were also dependent on the treated amount of KRG extract, and the SI-KRG50 group showed the most significant attenuation. The CRP concentration in the SI-KRG50 group was especially similar to that in the control, suggesting that KRG exerted efficient effects against stress-induced inflammation. In contrast, the treatment with 5 mg/kg/day of KRG (SI-KRG5 group) resulted in a marginal effect or did not elicit prominent parameter changes. These results, including the mitigated lipid levels, parallels the previous suggestion, indicating that KRG administration, combined with exercise, inhibited the elevation of TG, TC, LDL-C, and CRP levels in rats with atherosclerosis induced by a high-fat diet [28]. Similarly, ginseng supplementation significantly reduced CRP levels in human patients with a high baseline serum CRP value [29]. Besides, the treatment with 50 mg/kg/day of KRG restored the decreased insulin concentration, which was even higher than those in normal mice. However, KRG treatment showed little effect on TG concentration, which was not consistent with the previous report suggesting that serum TG level was decreased by the administration of KRG in humans [30]. Despite these discrepancies, the overall results suggest that KRG ameliorated the stress-induced detrimental indices, including pro-inflammation in plasma, in an extract concentration-dependent manner.
In addition to alleviating the production of hormones, lipids, ROS, and CRP in plasma, KRG administration impeded ROS formation in the liver of SI mice (Fig. 1A). As with the results shown in Table 1, the inhibition was dependent on the administrated amount, which was the most remarkable in the SI-KRG50 group. The decreased ROS level was also prominent in SI-KRG20 group, indicating effective anti-oxidative stress function of KRG. Fig. 1B also shows that KRG treatment inhibited the catalytic activities of CYP1A2 and CYP3A4 in the microsomes of SI mice, which were similar values to normal mice in the SI-KRG50 group. When qRT-PCR was performed to correlate these inhibitions of CYP activities with gene expression (transcription), KRG exhibited little effects: the down-regulation of CYP1A2 was shown only in the SI-KRG50 group, and the expression levels for CYP3A4 and CYP2E1 were not changed in all SI-KRG groups (Fig. 1C). Therefore, it may be a rational inference that the relation between KRG and CYP gene expression is currently unclear. Moreover, it is also uncertain whether the component(s) in KRG directly inhibits the enzyme activities or whether KRG-caused signaling pathways are involved in the inhibitory effect. Since CYP1A2 and CYP3A4 can generate ROS as mentioned, the diminished ROS generation shown in Fig. 1A may result from the KRG-mediated inhibition of the enzyme activities. Likewise with no noticeable effect of the stress on CYP2E1, all KRG treatments resulted in the similar enzyme activities to those in the control.
DISCUSSION
The present study provides results for stress-induced changes in plasma lipid and hormonal parameters, including ROS and CRP, and CYP activity-derived oxidative stress in the liver of mice. Collectively, the overall results are consistent with previous studies [22], implying that the stress negatively affected plasma and the liver in mice. However, some analyses showed differences with the reported suggestions demonstrating that stress increased glucose level and decreased TG concentrations in a bird species [31]. Moreover, regarding insulin, the relationship with stress is more complicated, and the reported results are conflicting with each other. Some studies suggested that plasma insulin levels increased in CORT-treated animals [32], whereas it was reported that cortisol directly suppresses pancreatic insulin release and also reduces glucagon-like peptide-1 production [33]. Furthermore, acute and chronically stressed rats showed different insulin secretion from isolated pancreatic islets [34]. Therefore, the relation between stress and insulin concentration is still unclear, which should be further revealed at molecular levels. It may be deduced that these discrepancies are ascribed to using different stress models and species, including various stress intensities. Even feeding status in animal models may affect stress-induced outcomes [34]. In addition to these factors, other experimental variables, such as gender, the timing of stress induction, and handling consistency across test groups are also possible to influence the stress-related parameter changes and therefore be important in obtaining consistent results. By controlling the variables and the standardization, more reliable studies on stress may be undertaken.
The present results suggest that the stress increased the activities of the CYP tested, while the expression levels did not change. Regarding the relation between stress and CYP expression, the effects of stress on CYP1A regulation have been reported to be stress-specific and species-specific [35]. Therefore, the molecular mechanisms, such as signaling pathways for the stress-mediated regulation of CYP1A expression, remain unclear. Furthermore, the stress-specific and species-specific responses may be confounded by the fact that even the same individual may respond to the same stress differently according to intrinsic and extrinsic conditions [35]. Although it has been known that the up-regulation of CYP3A is mediated by the main effectors of stress, epinephrine and CORT [36], the relationship between stress and the gene regulation of CYP3A has also yet to be verified; early maternal deprivation and restraint stress induced up-regulation of CYP3A1/2 expression in the liver of adult rats and in the murine liver, respectively, while exposure of adult rats to repeated restraint stress had no significant effect on CYP3A1 [36]. Despite all these controversies, it was deduced from the current study that the stress stimulated CYP1A and CYP3A4 activities, and consequently, the ROS generation was elevated in the liver. Since CRP may induce ROS production and alter the antioxidant defense system in endothelial cells [37], the stress-mediated CRP increase may elicit the increased ROS formation in plasma, although the origins and molecular mechanisms for ROS production in the blood are diverse.
KRG treatment decreased the elevated levels of CORT, TC, LDL-C, ROS, and CRP, and restored the insulin concentration in the plasma, compared to the SI group. The treatment remarkably diminished the enhanced CRP concentration and ROS, elucidating the anti-inflammation and anti-oxidative stress effects of KRG. As mentioned that ROS acts as an inflammatory response trigger [25], KRG-caused attenuation in CRP levels may explain the concomitant ROS reduction. However, the mechanism(s) through which KRG suppresses the CRP expression at the gene level could not be inferred. Conversely, the possibility of regulating CRP expression by ROS could not be also excluded; it was demonstrated that mitochondrial ROS affect on the transcriptional promotion of pro-inflammatory cytokines [38]. However, direct up-regulation of CRP by ROS has yet to be reported. Based on these results, the inhibitory effects of KRG on ROS formation can be responsible for the restoration of enhanced CRP. Taken together, these results suggest that KRG abates the detrimental stress-induced effects of hormones and lipids, as well as inflammation, in mice plasma, although some parameters were marginally changed. However, the effects of KRG on the stress-related parameters are not always consistent with the previous reports. For example, in contrast with the present result, for example, it was suggested that KRG induced an increase in TG levels in human, which was associated with an epinephrine decrease, implying that KRG may stabilize the sympathetic nervous system [14]. KRG also exhibited no effect on the insulin concentration in humans under stress conditions, compared to placebo treatment [39]. Therefore, when collectively judged by the previous studies, it is considered that the effect of KRG on anti-stress responses may depend on species, stress types, stress intensities, and SI conditions. Besides, other conditions, such as feeding environments may play important roles in the stress-responsive results of KRG. Nonetheless, it is widely accepted that KRG has beneficial effects on various animals under stress, including humans.
Although several studies have evaluated the interaction of KRG with the activities of major CYP enzymes, including their expression levels, each result is still conflicting. The activities of CYP1A2, CYP2C9, and CYP3A4 were inhibited by RG products in healthy humans [40], whereas no clinically significant drug interactions were observed between RG and these CYP enzymes [41]. In addition, many controversial results have also been reported regarding the efficacy of RG against other CYP enzymes in experimental animals. Although further studies should be undertaken to reveal the signaling pathways and/or effective components in KRG, the current results, for the first time, suggests the inhibitory effect of KRG on CYP1A and CYP3A4 enzymes and the resultant attenuation of ROS formation. It should also be noticed that the anti-stress effects of KRG were the treatment-concentration dependent. The exerted efficacy was the most prominent in the SI-KRG50 group, while the administration with 5 mg/kg/day of KRG (SI-KRG5 group) extract elicited little increase or decrease in the parameters tested. However, the results do not imply that the treatment of 50 mg/kg/day was the most effective for ameliorating the stress effects. Therefore, the experiment should be undertaken with a more diverse concentration range of the extract to determine the optimal dose.
In conclusion, the current study suggests that KRG extract may be applied to clinical fields to recover or protect stress-imposed body conditions in animals. Therefore, further studies should involve the functional mechanisms of KRG for anti-stress in more detail, the optimum dosage, and possible side effects in species-specific and stress-specific ways.