INTRODUCTION
Endocrine disrupting chemicals (EDCs) are a global environmental and human health problem that may pose a threat. They are substances in our environment, food and consumer products that interfere with hormone biosynthesis, metabolism or action, resulting in a deviation from normal homeostatic control or reproduction [1, 2]. The expanding production of synthetic chemicals has drawn attention to endocrine-disrupting compounds like bisphenol A (BPA). BPA is a widely recognized endocrine disruptor and pervasive environmental contaminant. Humans are continually and persistently exposed to BPA primarily through contaminated food and beverages, raising concerns about its potential for bioaccumulation and associated health risks [3]. The intestine, a critical organ for digestion and nutrient absorption, is particularly vulnerable to BPA exposure. Extensive animal studies have demonstrated significant intestinal changes due to BPA, including disruption of barrier function, altered nutrient absorption, and changes in the microbiome [4, 5]. Researchers make use of the HT-29 cell line, which expresses important receptors and has been used to investigate the effects of BPA on intestinal cells. This allows them to have a deeper understanding of these affects [6–8]. In vitro investigations that make use of two-dimensional (2D) cell cultures constitute the majority of the groundwork for biological research. Despite the fact that the 2D cell culture method continues to be a major methodology in research, the inherent constraints of this approach have led to the development of models that more accurately replicate the environment that exists in vivo [9]. Despite the fact that efforts have been made to overcome the restrictions of 2D cell culture, there are still limits to 2D cell culture that continue to exist. 2D cell culture based experiments cannot represent the in vivo environment and the outcome of these characteristics, which include the absence of polarity and the inability to self-organize into higher-order tissue structures. Therefore, developing methods for three-dimensional (3D) cell culture that can represent organic characters and functions is needed [10]. In recent years, 3D cell culture techniques have developed as a potentially useful tool for bridging the gap between animal models and cell culture technologies. Furthermore, the 3D spheroid culture method is mostly utilized as a straightforward and widely accepted model for the purpose of drug screening in the field of cancer research [11].
Organoids are 3D structures representing the homeostasis of self-organizing stem cells and their derivatives. These organoids can replicate a 3D microenvironment, which is believed to enhance the relevance of cell models by more closely mimicking in vivo conditions [12, 13]. Additionally, intestinal organoid (I/O) models currently provide researchers with effective tools for toxicity assessment and drug screening, allowing for the accurate evaluation of toxicity or efficacy [14, 15].
Altogether, the effects of BPA on the intestine have been extensively studied in vivo, demonstrating significant morphological and functional alterations. However, research investigating the impact of BPA on I/Os remains limited. In the current study, a more accurate functional and morphological changes in the I/O studies brought by BPA exposure was investigated in contrast to the conventional 2D cell cultures.
MATERIALS AND METHODS
The HT29 human cell line was purchased from the Korean Cell Line Bank (KCLB, Seoul, Korea) and cultured in 100 mm cell culture dishes in Dulbecco's modified Eagle’s medium (DMEM, Thermo fisher Scientific, Waltham, MA, USA) containing 10% fetal bovine serum and 1% penicillin/streptomycin. The medium was replaced every 2 days. HT-29 cells were maintained at 37°C in a 5% CO2 incubator. For cell treatments, HT-29 cells were used between passages 3 and 10. BPA and Estradiol were purchased from Sigma-Aldrich (St. Louis, MO, USA). BPA and Estradiol were dissolved in 100% DMSO, then diluted with a cell culture medium to final concentration 0.1 μM, 1 μM, 10 μM and 100 μM. Estradiol 1 nM was used as a positive control group.
HT-29 cells (1 × 104 cells/well) were seeded on 96-well cell culture dish and cultured in cell culture media for 24 hr, then treated with of Estradiol 1 nM and different concentrations of BPA (0.1, 1, 10, and 100 μM) for 24 hr. After that, culture medium was replaced immediately by fresh medium, then cell counting kit-8 (Dojindo Laboratories, Kumamoto, Japan) was added into the medium in proportion. After 1 hr incubation, OD value of the medium was detected at 450 nm by Epoch Microplate Spectrophotometer (Agilent, Santa Clara, CA, USA). Each experiment was repeated three times.
Crypts were isolated and cultured as previously described [16]. Briefly, proximal small intestine sections of approximately 20 cm were harvested, opened longitudinally, cut into 2–4 mm pieces, rinsed with cold Phosphate-buffered saline (PBS) and incubated in 2.5 mM EDTA at room temperature for 15 min with gentle shaking. Crypts were released after vigorous shaking in cold PBS and passed through a 70 μM cell strainer (Corning, Corning, NY, USA) for further enrichment [12]. Approximately 300 crypts were embedded in a DMEM (Thermo fisher Scientific) and Matrigel (Corning) at a 1:1 ratio in 48-well plates. The organoids were incubated in a CO2 incubator for 30 min, and 250 μL I/O culture medium (StemCell Technologies, Vancouver, BC, Canada) containing 1% penicillin/streptomycin (Thermo fisher Scientific) was added. The organoids were then incubated at 37°C in a 5% CO2 incubator. The cell culture medium was changed every 2.5 days. To assessment by BPA exposure effect in treatment group, organoids were incubated with Estradiol 1 nM and different concentrations of BPA (0.1, 1, 10 μM) for 24 hr. To determine morphological changes, I/O media was then discarded, and the organoid cells were fixed using 2% paraformaldehyde for 15 min at room temperature. Representative photographs were obtained using Cytation 5 (Agilent). The Institutional Animal Care and Use Committee of Jeju National University approved all experimental procedures (approval number: 2022-0055).
Total RNA was extracted with TRIzol reagent (Thermo fisher Scientific) according to the manufacturer’s protocol and stored at –50°C until use. The concentration and quality of the RNA were measured by using Epoch Microplate Spectrophotometer (Thermo fisher Scientific). Subsequently, 1 ug of total RNA was used to generate the first-strand cDNA. The primers for the target genes were designed using NCBI Primer-blast (https://www.ncbi.nlm.nih.gov/tools/primer-blast/) for qPCR. Quantitative real time PCR (qRT-PCR) was performed with the QuantStudio 1 Real-Time PCR system (Thermo fisher Scientific) using Power SYBR™ Green PCR Master Mix (Thermo fisher Scientific). Relative gene expressions were determined using the comparative Ct method and normalized by GAPDH gene expression levels. Gene mRNA levels were expressed as the fold change relative to the mean value of the control group.
Results are expressed as means ± S.D. Statistical significance was assessed by Student t test or ANOVA followed by Bonferroni post hoc test for multiple comparisons when appropriate using Prism 4 software (Graphpad Software, Boston, MA, USA). A p value<0.05 was considered significant.
RESULTS
We first assessed the effects of exposure to different concentrations of BPA on HT-29 colon cancer cell line. HT-29 cells were treated with 0.1, 1, 10 μM BPA and 1 nM Estradiol for 24 hr, and then cell viability was measured. The concentration-dependent effect of BPA on HT-29 cells is presented in Fig. 1. Exposure to the concentration of BPA (10 μM) did not significantly affect cell viability, although cell proliferation was increased at overall concentrations compared to control cells in the absence of BPA. The smallest increase in cell proliferation was observed at the lowest concentration of 0.1 μM (Fig. 1).
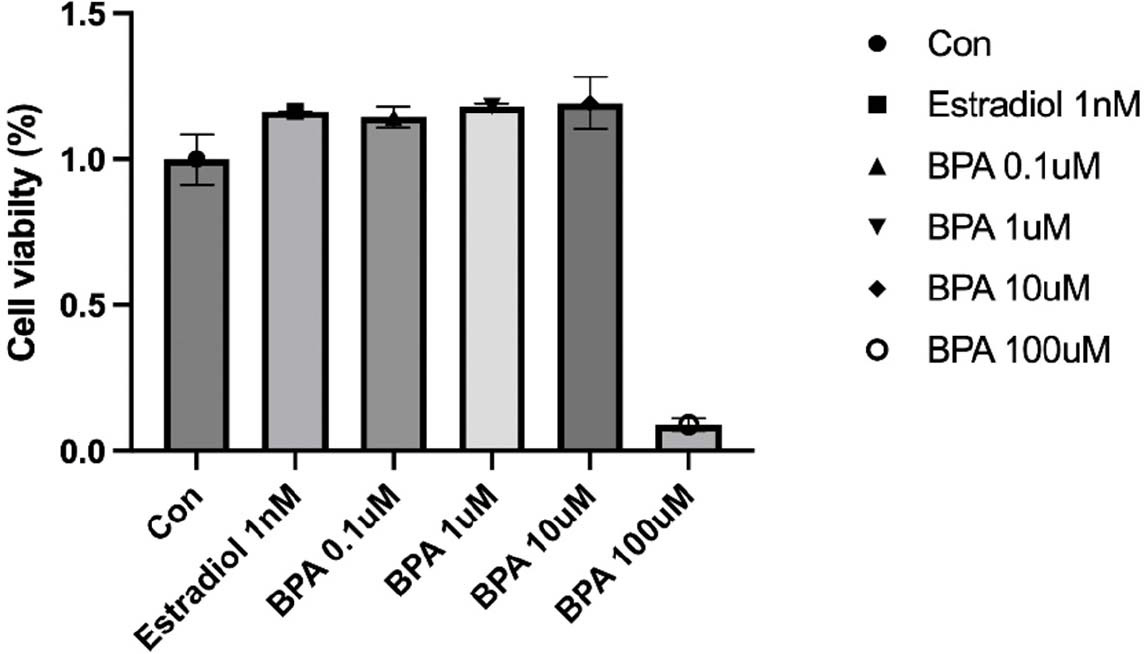
We performed to investigate the effects of BPA treatment on I/Os. Generally, Organoid cells begin to develop lumens and budding structures at around day 3–5 in culture. Therefore, we initiated BPA treatment after day 3, once organoid formation had commenced. Estradiol was used as a positive control for BPA treatment. As shown in Fig. 2A, budding structures expanded substantially during one day of culture in the presence of 1 nM Estradiol and at all concentrations of BPA compared to the control group. Additionally, the number of buds revealed that BPA 10 µM group exhibited a level of budding comparable to that observed in the estradiol-treated group (Fig. 2B). These results indicated that BPA induced morphological alteration. Alterations in organoid morphology were correlated with specific toxic effects and changes in intestinal function, including reductions in cell viability and differentiation, induction of apoptosis, dysfunction of mucus production, and damage to the epithelial barrier upon repeated administration [17].
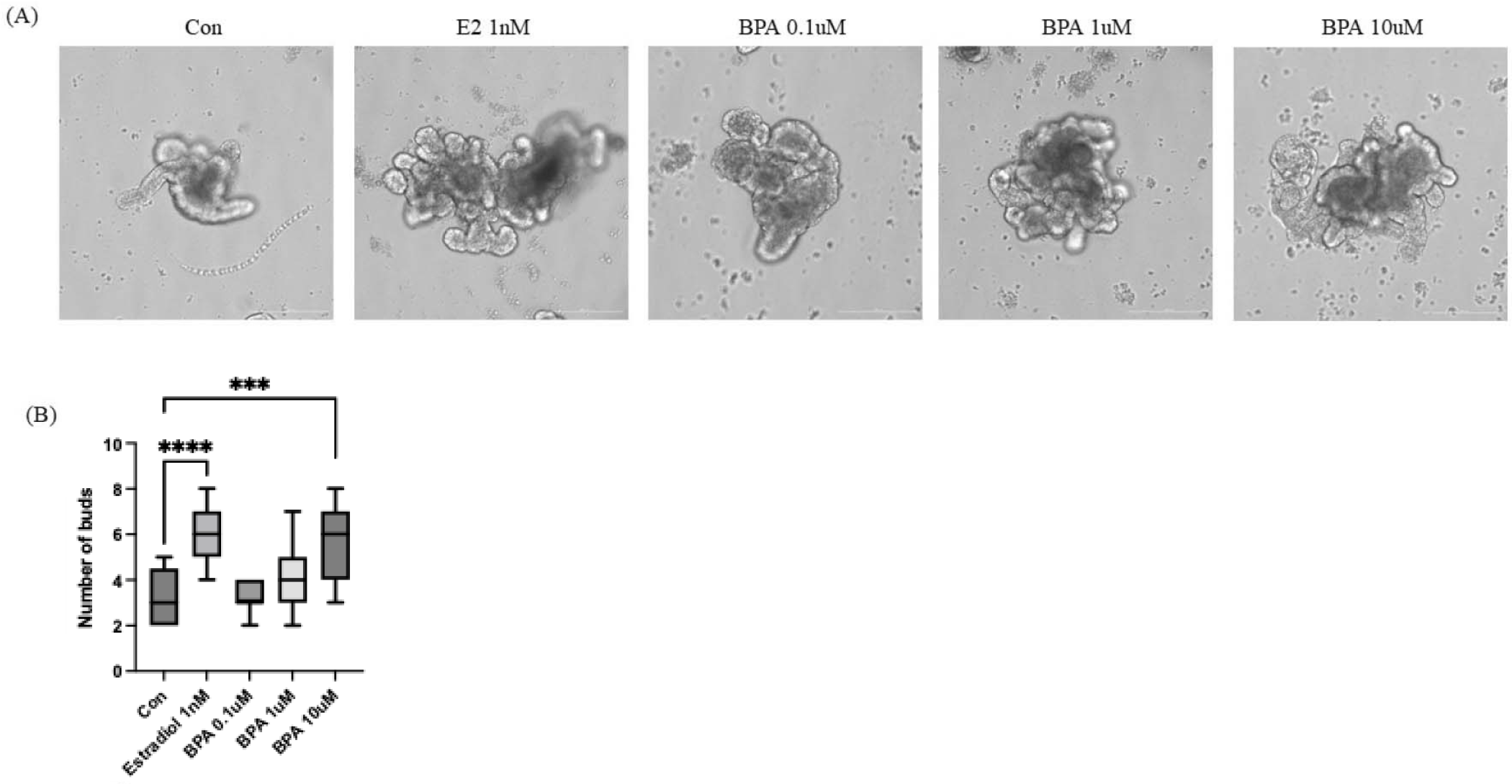
To determine whether BPA affects the proliferation of HT-29 cells and organoids, we initially examined the expression of key genes involved in the cell proliferation pathway. As shown in Fig. 3, HT-29 cells and I/Os treated with BPA showed an increased expression of PCNA, AKT, and ERK, which are important for cell proliferation, respectively. Notably, A significant increase in gene expression was observed 12 hr after BPA treatment. BPA is known to function as a xenoestrogen, exerting estrogen-like activity by interacting with classical estrogen receptors (ERα and ERβ) as well as the G protein-coupled receptor (GPR30/GPER) [18]. This compound can trigger diverse and divergent estrogen-signaling pathways, and to endorse tissue-specific, as well as developmental effects, including cellular proliferation and gene expression [3]. Previous research has shown that BPA increased the proliferation and migration of HT-29 colon cancer cells by activating the ERK signaling pathway [7]. Furthermore, it has been established that isolated mouse intestinal epithelium, when cultured as organoids, expresses detectable levels of ERβ and G protein-coupled estrogen receptors, with estradiol promoting organoid formation [19]. In this way, treatment with BPA similarly induces increased cellular proliferation in I/Os. Altogether, our results were consistent with previous experiments.
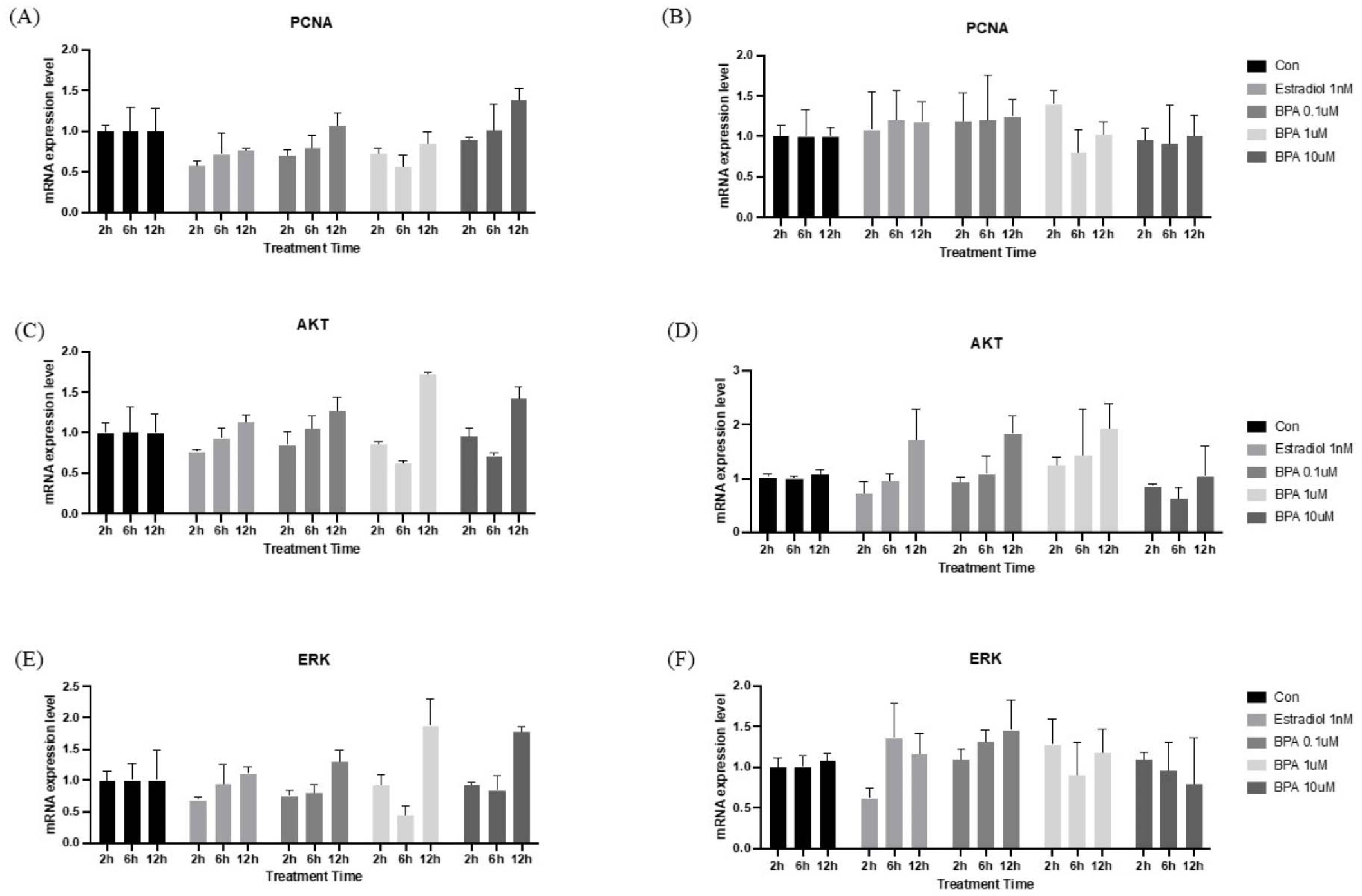
Alterations in intestinal permeability are increasingly recognized as being closely associated with gut barrier function. Tight junctions (TJs) are crucial components of gut barrier, consisting of multiple protein complexes located at the apical ends of the lateral membranes of intestinal epithelial cells. In mice, dietary BPA intake has been shown to disrupt the colonic physical barrier and increase gut permeability [20]. When comparing HT-29 cells and I/O mRNA expression level, it revealed a similar tendency in both groups. However, mRNA expression in I/Os was more pronounced than that of TJs in HT-29 cells. As shown in Fig. 4A, the expression of ZO-1 was observed to decline at 6 hr after BPA treatment in both the HT-29 and I/O groups. This decline was observed at the six-hour mark and subsequently reversed at the 12 hr. The mRNA expression of Occludin exhibits a comparable expression pattern between the two cultures methods (Fig. 4B). However, upon examination of each treatment group individually, mRNA expression in I/Os is more pronounced, particularly in the BPA 1 μM treatment group, where a notable decline in expression can be observed at the six-hour mark. As previously described, the overall mRNA expression patterns in the HT-29 and I/O groups were found to be similar. Despite the large error bars, the BPA treatment-induced expression of Claudin-2 and -4 in I/Os was observed to be comparable to that of the positive control (Fig. 4C and D). Nevertheless, previous studies have demonstrated that TJ mRNA expression is down-regulated in high concentrations of BPA [20, 21]. It is therefore evident that gene expression analysis is insufficient for determining correct expression level whether gene expression is up-regulated or down-regulated. A quantitative analysis of protein is essential, as mRNA expression does not indicate whether the product is translated into protein.
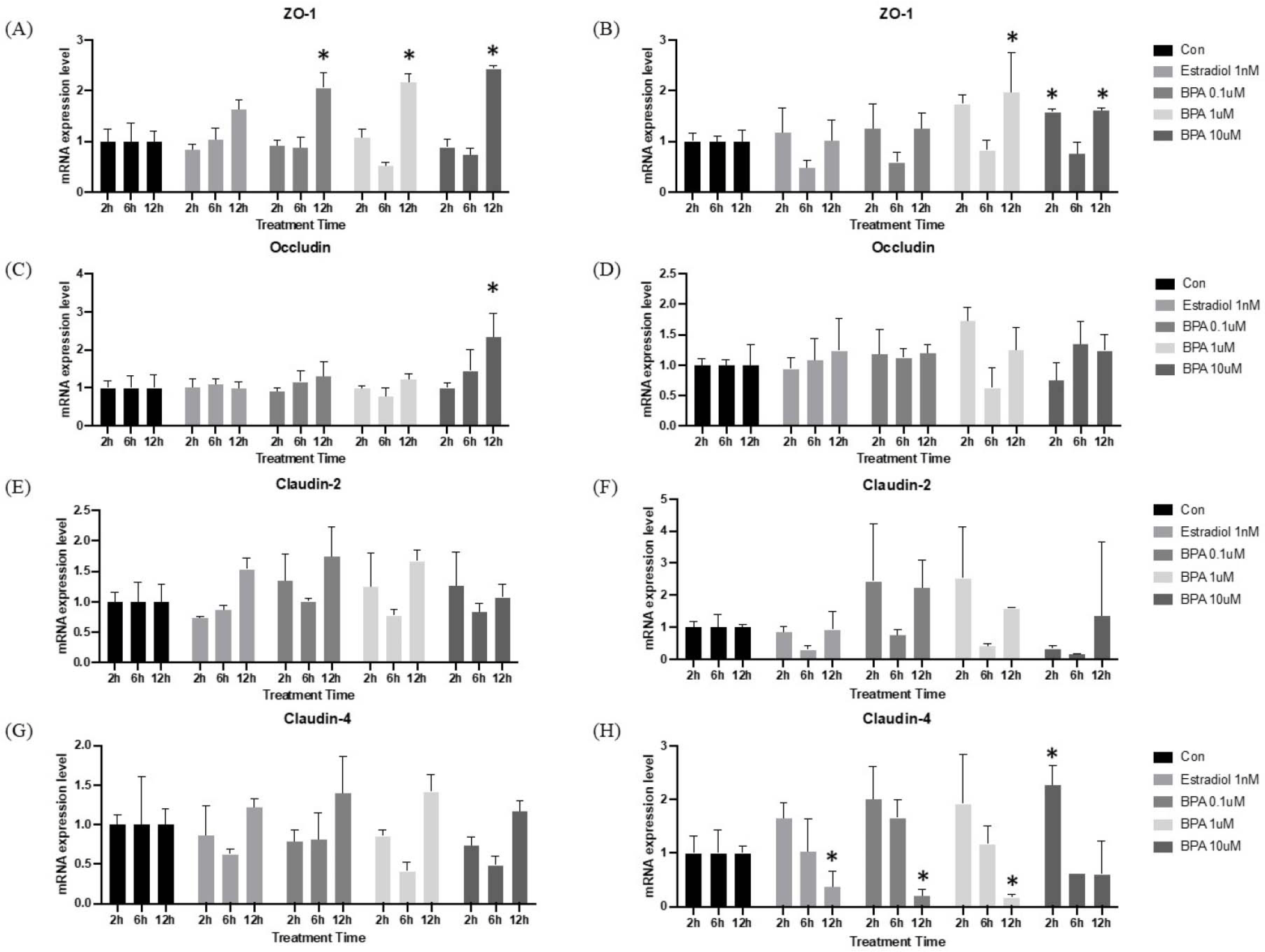
DISCUSSION
The aim of these experiments was to investigate whether there are differences between traditional 2D cell culture and 3D organoid culture. Furthermore, we investigated gene expression level following treatment time. BPA is widely acknowledged to be an EDCs and can be introduced into our body through exposure to storage and packaging materials. While the effects of BPA exposure on the gut have been studied both in vitro and in vivo, the I/Os have yet not been investigated. Therefore, a comparative analysis was conducted to investigate the effects of BPA on HT-29 and I/O models. Previous research has demonstrated that BPA is highly toxic to the intestine and causes intestinal barrier dysfunction at concentrations of BPA exceeding 100 µM [21]. However, when assessing cell viability on HT-29 cells, cells were not survived at exceeding 100 μM. Therefore, to examine the effects of BPA exposure in the gut, this study was conducted at a concentration below that which killed the cells. In general, the mRNA expression patterns observed in I/Os were comparable to those seen in the HT-29 cells. However, when examining genes that may indicate functional changes in the small intestine, such as TJs, the mRNA expression changes were more pronounced in the organoids than in the HT-29 cells, which are a 2D cell culture. This is regarded as advantages of 3D cultures, which have the capacity to expand in all directions, compared to conventional 2D cultures, which are formed as a monolayer. Previous studies have observed that the expression of TJ proteins is altered in patients with ulcerative colitis and inflammatory bowel disease [22, 23]. And even when humans and animals have been exposed to high or long-term levels of BPA, it can cause gut disorders such as microbiome dysbiosis, obesity and type II diabetes, and metabolic disorders [24–26]. These findings indicated that compromising TJs resulted in an increase in intestinal barrier permeability, which reflects decreased epithelial barrier function. In this case, the down-regulation of TJ expression was associated with the development of gut dysfunction, which is commonly referred to as leaky gut [27–29]. However, our data indicated that while the gene expression level linked with cell proliferation was up-regulated, the expression of genes associated with TJs was observed to decline at 6 hr after BPA treatment and subsequently reversed at the 12 hr. Furthermore, Basal-out I/Os have the basal membrane of the intestine on their surface. However, practical limitations exist for their potential use, such as challenges in chemical penetration, host-pathogen interactions and nutrient absorption, because organoids cultivated in Matrigel exhibit only basal-out structures [30, 31]. With the use of two models, namely the HT-29 colon cancer cell line and an intestine organoid model, as well as morphological alterations of I/Os, we investigate the impact that BPA has on the proliferation and TJ gene expression. However, at the same dosage, BPA does not result in a substantial increase in the number of HT-29 cells accordingly. The proliferation was boosted in a dose-dependent manner with I/O. With the exception of Claudin-4, the expression pattern of genes associated to proliferation and TJs was comparable across HT-29 and I/O. Based on these results, this research provide a more accurate portrayal of the functional and morphological changes that are brought about by BPA in compared to the conventional 2D cell cultures. Although further research is essential, this study is meaningful in that it provides a comparison between 2D and 3D organoid cultures with BPA treatment.