INTRODUCTION
Hyperadrenocorticism (HAC) is a common canine endocrine disease arising from the overproduction of cortisol by the adrenal gland. Clinical manifestations include a range of symptoms, such as an abdominal distension, polydipsia, polyphagia, polyuria, comedones, alopecia, and lethargy [1]. Prolonged excess cortisol leads to various complications, including congestive heart failure, pyelonephritis, steroid hepatopathy, systemic hypertension, cystic calculi, proteinuria, pancreatitis, diabetes mellitus, pituitary macrotumor syndrome, degenerative myopathy, and pseudomyotonia [2]. Of particular concern is hypercoagulability, with its potential association with pulmonary thromboembolism (PTE) [2, 3], and thromboembolic disease is one of the most serious complications associated with HAC in dogs [4].
PTE involves obstruction of pulmonary blood vessels by thromboembolism [5], representing a critical concern in cases of HAC. However, it is difficult to predict PTE in dogs because of challenges in ante-mortem diagnosis [6] and the rapid dissolution of thrombi postmortem [7]. Rudolph Virchow's conceptualization in the 1800s underscores three conditions contributing to pathological thrombus formation: blood flow stasis, endothelial damage, and hemostatic imbalance favoring coagulant factors (hypercoagulability) [4]. Among these conditions, hemostatic imbalance involves platelet hyperaggregation, excessive activation or decreased removal of coagulation factors, deficiencies of anticoagulants, or defective fibrinolysis [8].
Traditional plasma-based coagulation assays, including antithrombin activity, prothrombin time (PT), activated partial thromboplastin time (aPTT), fibrin degradation products, D-dimer, and fibrinogen, have limitations in assessing the overall hemostasis [4, 9-11]. Thromboelastography (TEG), which offers a comprehensive evaluation of platelet function, coagulation proteases, coagulation inhibitors, and fibrinolysis in whole blood, has emerged as a promising diagnostic tool [12, 13].
We hypothesized that dogs with HAC would exhibit greater hypercoagulability than healthy dogs. This study explored the use of TEG as a diagnostic tool for identifying hypercoagulability in dogs with HAC. This study’s results are expected to improve our understanding of hypercoagulability in dogs with HAC and elucidate the potential utility of TEG in diagnosing and assessing coagulation disorders.
MATERIALS AND METHODS
In this retrospective analysis, we evaluated coagulation parameters in dogs with HAC and healthy dogs. Medical records were obtained from dogs visiting Gyeongsang National University between 2018 and 2022. The inclusion criteria were dogs diagnosed with HAC on the basis of laboratory findings and diagnostic test results, including adrenocorticotropic hormone (ACTH) stimulation or low-dose dexamethasone suppression test (LDDST) results. The experimental group consisted of dogs with HAC and the control group consisted of healthy dogs.
Data, including history, physical examination findings, clinical signs, blood and urine test results, coagulation test results, and clinical diagnoses, were collected from the dogs’ medical records.
Blood samples were collected from the jugular or medial saphenous veins using a 23-gauge (G) needle or 24-G intravenous catheter. The samples were stored in ethylenediaminetetraacetic acid and lithium heparin tubes for blood examination, and citrate tubes for coagulation assessments. Urine samples were collected via cystocentesis or urinary catheterization.
Laboratory tests were performed on all the dogs to determine their condition. Blood pressure (Parks Doppler 811-B®, Parks Medical Electronics, Aloha, OR, USA) was measured before collecting the blood sample. The patients were allowed to rest calmly for 10 minutes before the measurement, and five blood pressure measurements were performed to obtain the average blood pressure. Blood examination included the complete blood count (Procyte Dx® Hematology Analyzer, IDEXX, Westbrook, ME, USA) and serum biochemistry (Catalyst Dx® Chemistry Analyzer, IDEXX). Serum biochemical analyses included alanine aminotransferase (ALT), alkaline phosphatase (ALP), triglyceride (TG), cholesterol, and glucose levels. Urine examination included urine specific gravity (USG); Rhino® VET 360 Refractometer, Ametek Reichert, Depew, NY, USA). Coagulation examination included PT, aPTT (Coag Dx® Analyzer; citrate PT and citrate aPTT cartridges; IDEXX), fibrinogen (Manual; heat precipitation), and TEG (TEG 5000 Thrombelastograph® Hemostasis Analyzer, Haemonetics, Boston, MA, USA). The fibrinogen concentration was measured by heat precipitation [14]. The order was as follows: fill two hematocrit tubes with heparinized blood (tubes A and B), heat one tube to 56°C–58°C for 3 minutes (tube B). Tubes A and B were centrifuged in a micro-hematocrit centrifuge. The total solids in each tube were measured using a refractometer. The amount of fibrinogen was calculated using the following formula: fibrinogen = total solids in tube A (g / l) – total solids in tube B (g / l). Next, we determined the amount of fibrinogen. TEG was conducted [15] as follows. To collect a blood sample, evacuated tubes and 21-G or larger needles were used. Heparinized cups were used to prevent the use of exogenous heparin. Blood samples were collected in 3.2% buffered sodium citrate at a strict 1:9 ratio (final concentration, 10.8-mM citrate). Blood samples for TEG were maintained at room temperature for 30 minutes before analysis and were not be refrigerated. The TEG results were interpreted considering method-specific reference intervals.
Clinical data and coagulation assays were compared between dogs with HAC and healthy dogs using a non-parametric test. All statistical analyses were performed using SPSS Statistics software (version 25.0, IBM, Armonk, NY, USA). p-values<0.05 were considered statistically significant. All graphical analyses were performed using GraphPad Prism software (GraphPad Software, La Jolla, CA, USA).
RESULTS
Thirty-one dogs representing a diverse range of ages, weights, sexes, and breeds were enrolled. Among these, 21 dogs were categorized into the HAC group, and the remaining 10 comprised the control group.
The dogs with HAC included 7 males (7 intact) and 14 females (12 intact), with a mean age of 12.67 ± 2.34 years (range, 1–16 years). Nineteen dogs were purebred, whereas two were mixed-breed: Maltese (n = 9), Yorkshire terrier (n = 3), Shih Tzu (n = 2), Chihuahua (n = 1), Miniature Pinscher (n = 1), Pomeranian (n = 1), Poodle (n = 1), and Schnauzer (n = 1). All the dogs were diagnosed with HAC. Nine dogs (42%) were diagnosed using the ACTH stimulation test, and 12 dogs (58%) were diagnosed using the LDDST. The signalment characteristics of dogs with HAC are listed in Table 1.
The control dogs included 8 males (7 intact) and 2 females (2 intact), with a mean age of 6.4 ± 2.91 years (range, 1–16 years). Ten dogs were purebred: Beagle (n = 5), Maltese (n = 2), Chihuahua (n = 1), Poodle (n = 1), and Cocker spaniel (n = 1). All dogs were healthy and were diagnosed using the LDDST. The signalment characteristics of the control dogs are shown in Table 2.
Among the clinical variables analyzed, the monocyte, platelet, ALT, ALP, TG, and cholesterol levels were significantly higher (p<0.05) in dogs with HAC than in healthy dogs (Fig. 1). There were no significant differences in blood pressure, neutrophils, eosinophils, lymphocytes, hematocrit, glucose, or USG between dogs with HAC and healthy dogs (Table 3).
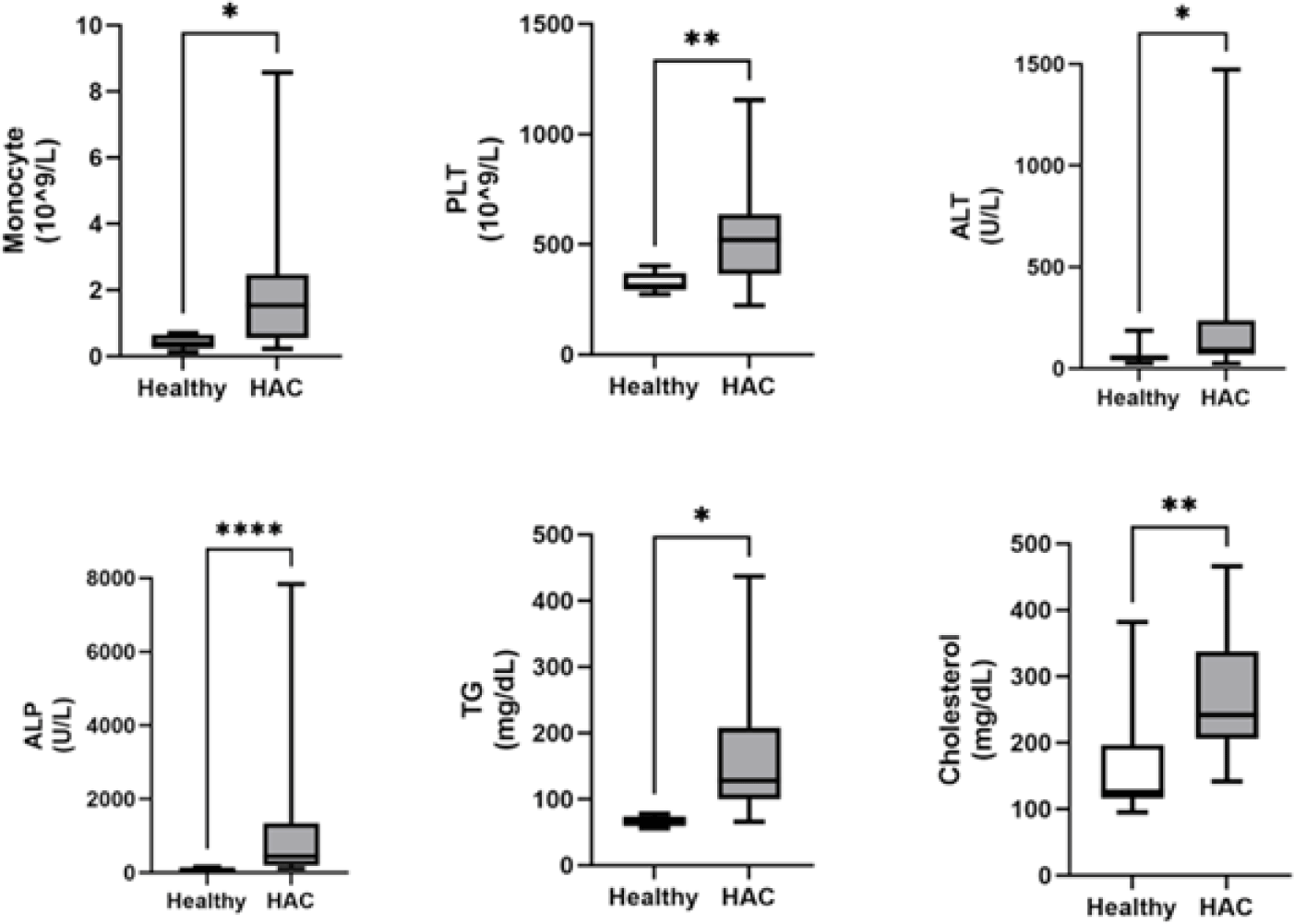
Traditional coagulation assays included PT, aPTT, and fibrinogen concentrations. For all three test results, there were no significant differences between dogs with HAC and healthy dogs (Fig. 2).
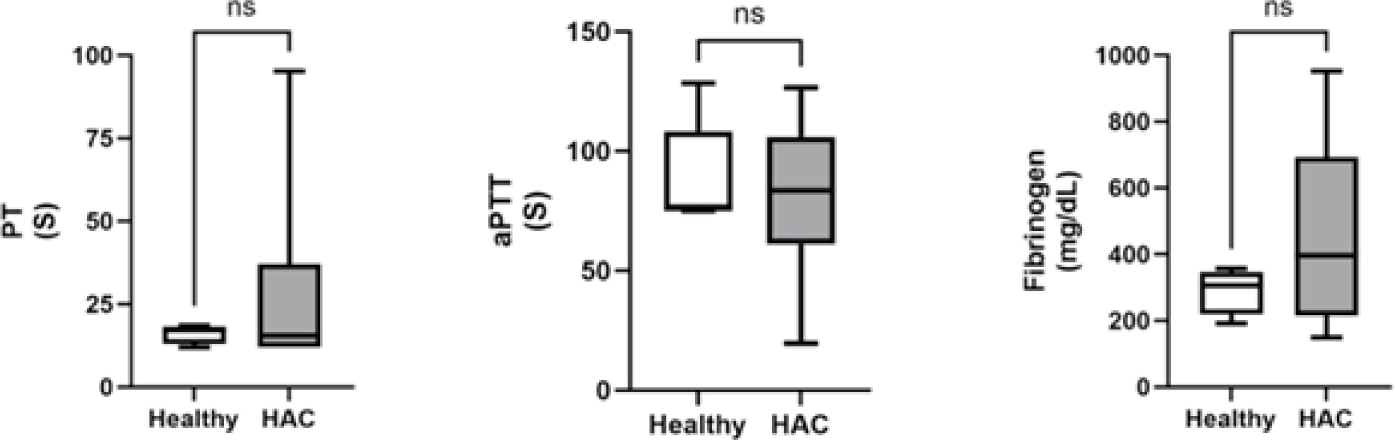
The K value was significantly lower (p=0.003) in dogs with HAC than in healthy dogs. The α and MA values were significantly increased in the dogs with HAC compared with healthy dogs (p=0.002 and p<0.001, respectively). There was no significant difference in the R values between dogs with HAC and healthy dogs (Fig. 3).
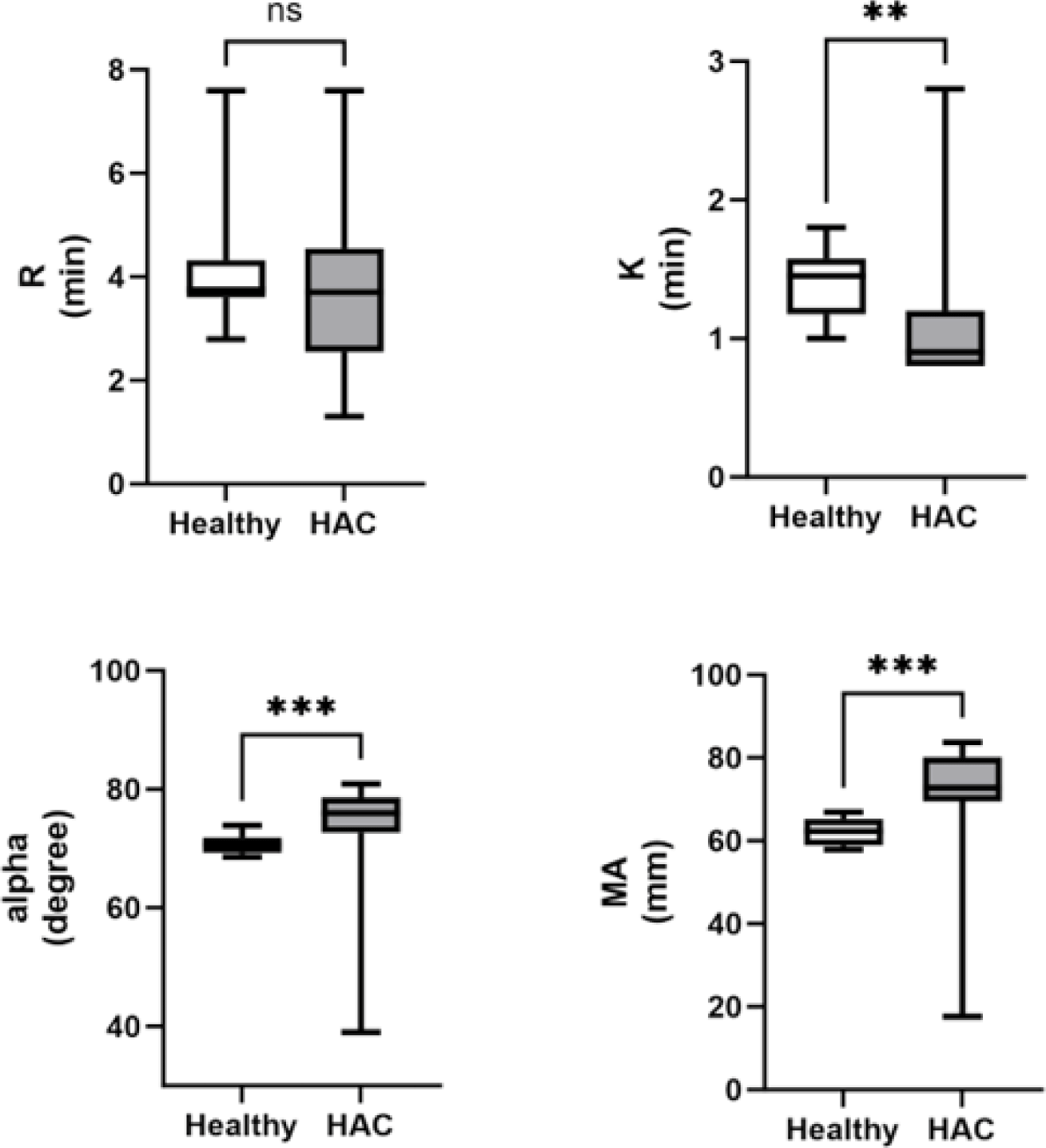
DISCUSSION
This study analyzed coagulation parameters, such as PT, aPTT, fibrinogen, and TEG values, to confirm whether dogs actually demonstrate hypercoagulability compared to healthy dogs. Additionally, conventional laboratory parameters, such as systolic blood pressure, blood examination results, and USG, were analyzed to confirm the clinical characteristics of dogs with HAC.
Dogs diagnosed with HAC face significant complications such as thromboembolism, even with trilostane treatment [2]. Thromboembolism can affect vital organs including the kidneys, gastrointestinal tract, heart, and central nervous system, potentially leading to PTE and respiratory distress. However, it is difficult to predict PTE in dogs because of challenges in ante-mortem diagnosis [6] and the rapid dissolution of thrombi postmortem [7].
The decrease in the K value and the increase in the α and MA values in dogs with HAC demonstrated in this study aligned with findings from previous research [16]. A similar tendency toward hypercoagulability in the HAC group (88.2%) was reported previously [17]. A hypercoagulable TEG tracing, characterized by decreased R and K values and increased α and MA values, indicates a shortened clotting time and enhanced strength of blood clot formation [18]. The assumption that hypercoagulability may be associated with excessive cortisol in the adrenal gland warrants further investigation into the factors influencing hypercoagulability in dogs with HAC. TEG has emerged as a valuable tool for identifying patients prone to blood clot formation (hypercoagulability) [19]. Although TEG does not elucidate the specific etiology of hypercoagulability in various clinical states, it enables the identification of a tendency toward hypercoagulability in individuals and specific populations [12].
Here, the R value exhibited a lower statistical significance than the K value. The R value, which represents the latency period from blood withdrawal to clot formation, is primarily related to plasma clotting factors. In contrast, the K value, which represents the time to clot formation, is primarily related to fibrinogen [11]. The combined R and K values provide insights into the coagulation time from initiation to a predetermined clot strength [19]. Although the fibrinogen concentration was assumed to have a more significant impact on clot formation than clotting factors in dogs with HAC, further research is needed to explore additional factors that may affect the R and K values in this context.
In the present study, a significant increase in the platelet count was found in dogs with HAC compared with healthy dogs. Thrombocytosis, defined as an elevated number of platelets in the peripheral blood [20], has been previously reported in dogs with HAC and in those receiving glucocorticoid treatment, although the underlying mechanism remains unclear [21]. Thrombocytosis observed in this study prompted further investigation of the intricate interplay between HAC and platelet dynamics. The MA value obtained from TEG analysis is a comprehensive assessment of the platelet count, function, and fibrinogen activity [22].
In our investigation, the MA value exhibited a noteworthy increase in dogs with HAC, aligning with findings from a recent study [23] wherein 96% of HAC-afflicted dogs demonstrated an elevated MA value. However, while 77% of dogs with HAC were found to be hyperfibrinogenemic in that particular study, no significant alterations were observed in conventional coagulation assays such as PT, aPTT, and fibrinogen concentration in our study. This suggests that the utility of employing TEG may be underscored compared to established coagulation assays when assessing hypercoagulability.
Furthermore, the k value fell below the reference interval in only 22%, and the alpha value exceeded the RI in only 35% of dogs with HAC in a prior study [23]. In contrast, our study identified significant changes in both k and alpha values among dogs with HAC. This suggests that these alterations could provide valuable insights for guiding future treatments based on the characteristics of hypercoagulability prior to the initiation of medical or surgical interventions in dogs with HAC. The increased MA value in TEG analysis suggested a potential correlation between platelet dynamics and hypercoagulability in dogs with HAC. Further research is warranted to delve into the specifics of this relationship and ascertain whether thrombotic events occur in dogs with HAC. Thrombocytosis in dogs with HAC, as demonstrated in our study, raises questions about its clinical significance and potential association with thrombotic complications. In human medicine, thrombocytosis is considered a prognostic factor for hemorrhage or thrombosis in certain disease states [24, 25]. By extending this knowledge to veterinary medicine, our findings highlight the need for future investigations to establish the correlation between platelet dynamics, as indicated by thrombocytosis and the MA value, and the occurrence of thrombotic events in dogs with HAC. This study elucidates the hematologic alterations in HAC, emphasizing the significance of platelet dynamics. Further research is essential to elucidate the mechanistic link between thrombocytosis, as observed in dogs with HAC, and the hypercoagulable state, which would offer valuable insights into the clinical implications of these hematologic changes in canine patients.
Leukogram analysis revealed a significant increase in monocytes in dogs with HAC compared with healthy dogs. Although not statistically significant, there was a tendency toward neutrophilia, lymphopenia, and eosinophilia. These findings align with those of a previous study, suggesting a consistent hematologic pattern known as a stress leukogram in dogs with HAC [26]. Monocytosis, which is attributed to capillary margination and diapedesis associated with excess glucocorticoids, supports the notion of a stress leukogram, implicating excessive cortisol secretion from the adrenal gland as a contributing factor.
Among the liver enzymes, both ALT and ALP levels were significantly increased in dogs with HAC compared with healthy dogs in this study, which is consistent with the findings of a previous study [27]. The increased ALT activities are believed to indicate liver damage, possibly due to glycogen accumulation in hepatocytes, as suggested in another study [28]. Elevation of the ALP level, observed in > 90% of dogs with HAC, is attributed to the induction of a specific hepatic isoenzyme by glucocorticoids, a phenomenon unique to dogs [26]. The observed increases in ALT and ALP levels were presumed to result from excessive cortisol secretion from the adrenal gland.
Significantly higher concentrations of TG and cholesterol levels were observed in dogs with HAC. Hyperlipidemia, defined as an increase in the serum TG or cholesterol concentration [29, 30], is a common condition in dogs and is either a primary or secondary disorder [31]. Secondary hyperlipidemia, which is more prevalent than primary hyperlipidemia, can be associated with endocrine disorders, e.g., hypothyroidism and HAC, among other factors, e.g., obesity, high-fat feeding, and corticosteroid therapy [32, 33]. The observed elevations in TG and cholesterol concentrations were hypothesized to be a consequence of excessive cortisol secretion from the adrenal glands of dogs with HAC.
Blood pressure tended to be higher in dogs with HAC than in healthy dogs, although the difference was not statistically significant. Systemic hypertension, which occurs in > 50% of untreated dogs with HAC, may result from various mechanisms, including the activation of the renin-angiotensin system and increased vascular sensitivity to catecholamines [26]. Mechanisms of hypertension potentially include excessive secretion of renin and decreased vasodilator prostaglandins [3]. The observed tendency toward high blood pressure was attributed to excessive cortisol secretion.
Although our study highlighted hypercoagulability in dogs with HAC using TEG, the lack of postmortem analysis and medical or surgical treatment in these dogs is a limitation of the study. Additionally, the study did not include other laboratory test results, such as fibrin degradation product, D-dimer, P-selectin, activated protein C, and antithrombin, warranting consideration in future investigations.
Our study demonstrated hypercoagulability in dogs with HAC compared with healthy dogs. Predicting blood clot formation in dogs is challenging owing to various contributing factors. Nevertheless, this study’s findings suggest the potential utility of TEG for predicting blood clot formation in dogs with HAC, emphasizing the need for further research in this area.