INTRODUCTION
Inflammatory conditions within the male reproductive tract could impair sperm quality, cause seminal tract obstruction, reduce androgen production, and affect spermatogenesis, thereby affecting normal reproduction and increasing the risk of infertility [1, 2]. Toll-like receptors (TLRs) can be widely found on the surface of monocytes, macrophages, and dendritic cells. TLRs play a vital role in pathogen recognition by the innate immune system, regulate immune responses, and mediate the production of inflammatory factors. Among the TLRs, TLR4 acts as a receptor for lipopolysaccharides (LPS). TLR4 binds to the myeloid differentiation protein 2 to form a complex that interacts with the LPS, which in turn triggers inflammatory responses [3, 4]. Obesity is associated with the upregulation of the TLR4 protein and its activation by LPS and free fatty acids triggers systemic inflammation seen in obesity and the metabolic syndrome [5, 6]. High-fat diet (HFD)-induced obesity can affect male fertility through several mechanisms including endocrine system regulation, scrotal temperature elevation, oxidative stress, and changes in ejaculatory functions, which can directly impair sperm production and /or functions [7, 8]. Several previous studies have shown that TLR4-deficient mice chronically fed with HFD are protected against inflammation and insulin resistance [9–11]. However, a direct correlation between TLR4 deficiency and male fertility in HFD-induced obesity remains unclear. Therefore, more research is needed to understand the effects of the TLR4-signaling pathway on male fertility in obese individuals. The present study was performed to examine the testicular function and fertilization capacity of TLR4 knockout (KO) male mice fed with an HFD.
MATERIALS AND METHODS
C57BL/6 (wild-type [WT]) mice were purchased from Samtako Bio Korea (Osan, Korea), and the TLR4 KO mice (TLR4) are described elsewhere [12]. Six-week-old WT and TLR4 male mice were fed ad libitum with a control normal chow diet (NCD) or a HFD that included 40% kcal from soybean and coconut oil (Research Diets, New Brunswick, NJ, USA) for 20 weeks, and then were sacrificed to collect serum and organs (Fig. 1). The animals were housed in a facility at 22℃–25℃ with a humidity of 50 ± 10% and a 12 hr light/dark cycle. All mice experiments were performed according to the Institutional Animal Care and Use Committee (IACUC) protocol guidelines and approved by the IACUC of Chungbuk National University, Korea (CBNUA-1626-21-02).
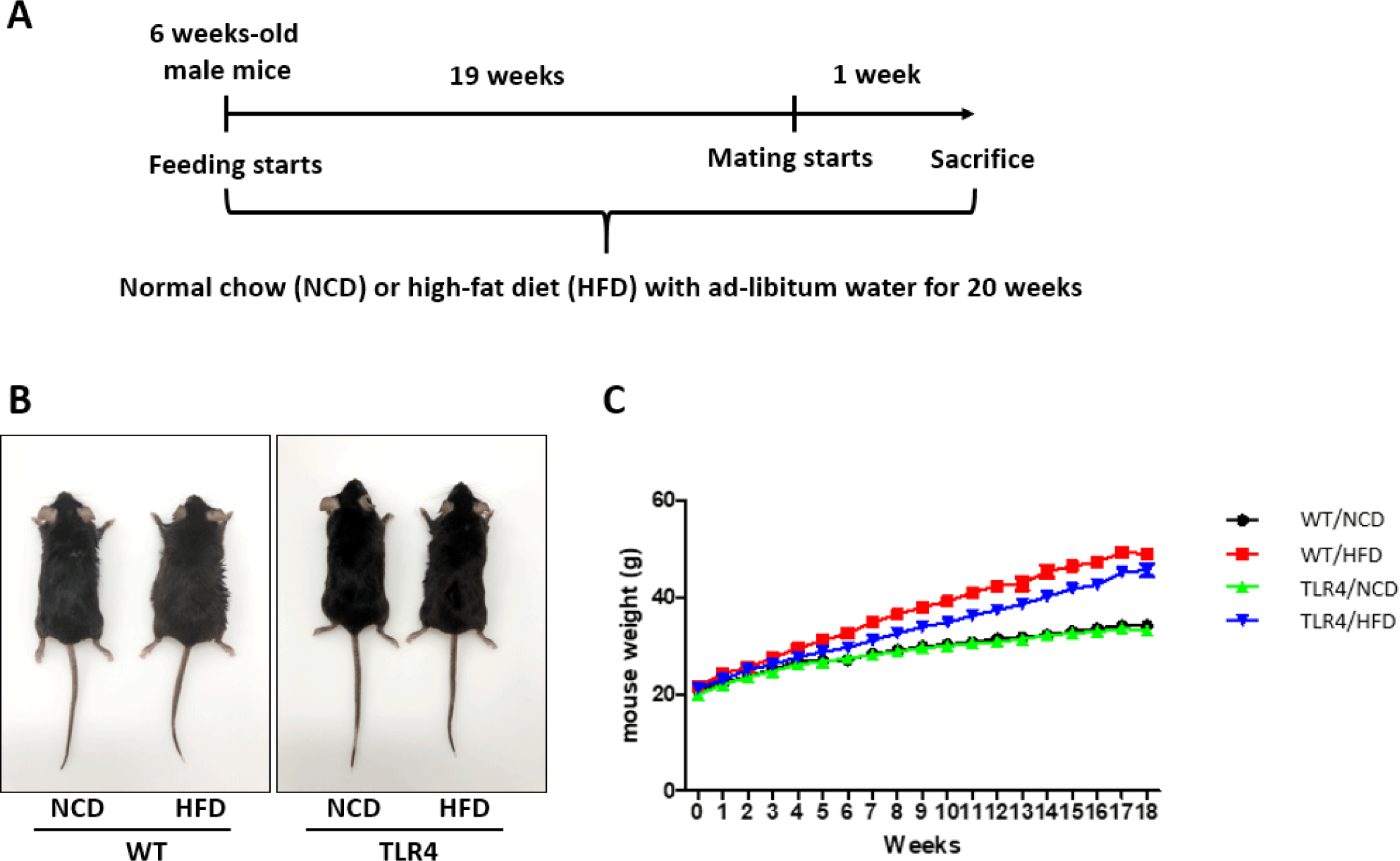
Unless otherwise stated, all other reagents used in this study were purchased from Sigma-Aldrich (St. Louis, MO, USA).
After the mice were fed with an NCD or HFD for 19 weeks, male mice were randomly selected from each group for mating. A female mouse was mated with a male mouse overnight. Subsequently, the presence of a vaginal plug in the mice was checked and designated as day 0.5 of gestation. Pregnancy rate and litter size were examined after delivery (Fig. 1A).
Carbon dioxide (CO2) inhalation was used to sacrifice the treated mice in all the groups. Blood was aspirated by a 1 mL syringe, and centrifuged at 500 × g for 10 min. Thereafter, serum was collected and stored at –20°C for further examination. The liver, testes, and seminal vesicles were excised and immediately weighed on an electronic scale. The dissected epididymis was washed thrice with phosphate-buffered saline (PBS). Afterward, it was minced in 2 mL PBS supplemented with 0.1% polyvinyl alcohol (PBS-PVA) using a surgical blade and kept at 37.5°C for 10 min. Then, the motility of the released sperm was estimated under a light microscope at 400× magnification. A hemocytometer (Marienfeld-Superior, Lauda-Königshofen, Germany) was used to estimate sperm concentrations.
The serum concentrations of glucose, cholesterol, and triglycerides, were measured using the respective kits (Asan Pharmaceutical, Seoul, Korea) according to the manufacturer’s protocol. The concentrations of glucose and cholesterol were inferred using the corresponding standards by measuring the optical density (OD) value at 500 nm, and the concentrations of triglycerides were assayed using corresponding standards by measuring the OD values at 550 nm (Absorbance 96, Byonoy, Hamburg, Germany).
Glutamate oxaloacetate transaminase (GOT) and glutamate pyruvate transaminase (GPT) were assayed by the Reitman-Frankel method [13]. The serum levels of the enzymes were determined using commercial detection kits based on the manufacturer’s instructions (Asan Pharmaceutical).
The motility of the isolated spermatozoa from epididymis was estimated using PBS-PVA under a light microscope at 400× magnification. Thereafter, sperm concentration was measured by using a hemocytometer (Marienfeld-Superior). It was reported that spermatozoa with a low concentration of proAKAP4/AKAP4 were categorized as abnormal staus [14]. Therefore, in order to measure the proAKAP4, the spermatozoa in PBS-PVA was centrifuged at 500 × g for 5 min, the supernatant was removed, and the pellet was used for analysis according to manufacturers' guidelines (4BioDx, Lille, France). Sperm samples were incubated with a detection antibody, A-kinase anchor protein 4 precursor (pro-AKAP4), and horseradish peroxidase substrate solution. Afterward, a stop solution was added immediately, and the OD was determined at 450 nm by a microplate reader (Absorbance 96).
The testis was fixed in 10% neutral-buffered formalin (Sigma-Aldrich). Thereafter, the fixed tissues were dehydrated in serial ethanol solutions (50%, 70%, 80%, 90%, and 100%) respectively, and cleared in xylene. Subsequently, tissues were embedded in paraffin which was sectioned to 5 μm thickness. Then, sections were deparaffinized from the series of xylene and rehydrated through serial ethanol (100%, 90%, 80%, and 70%), respectively. The tissues were then stained with hematoxylin for 5 min. Finally, the tissue sections were washed in distilled water (DW) and were counterstained with eosin for 15 min. A mounting solution (Sigma-Aldrich) was used to mount the tissue sections for further observation under the light microscope.
All experimental data were expressed as mean ± S.E.M. and analyzed using one-way ANOVA on the GraphPad PRISM® (GraphPad Software, San Diego, CA, USA). A completely randomized design was applied, and Tukey’s multiple comparison test was performed to compare the values of individual treatments. The results were considered statistically significant at p-values *p<0.05, **p<0.01, and ***p<0.001.
RESULTS
Morphological appearance of mice (Fig. 1B) and changes in body weight due to the NCD or HFD (Fig. 1C) were measured in all the experimental groups. With a significant accumulation of body fat in the HFD groups relative to their respective controls, the obesity profile of the HFD-fed WT and TLR4 mice was enhanced (Fig. 1B). The body weight of WT and TLR4 mice fed with a HFD significantly increased relative to their respective NCD fed controls through the experimental period (Fig. 1C).
Mice were sacrificed at the end of the 20th week after being fed with the HFD or NCD, and body weight, perigonadal fat weight, and organ weights including liver, spleen, and reproductive organs were measured (Fig. 2). Both WT and TLR4 mice fed a HFD showed significantly higher body weight compared to the mice fed a NCD (p<0.01 and p<0.001; Fig. 2A). The perigonadal fat weight was significantly higher in the TLR4 mice fed a HFD compared to the other experimental groups (p<0.05 and p<0.001; Fig. 2B). Higher weights of the liver and spleen were observed in WT mice fed a HFD compared to those of the WT and TLR4 mice (p<0.01; Fig. 2C and D). Testicular weights were not significantly different between the NCD and HFD-fed TLR4 mice. However, WT mice fed with a HFD had a significantly higher testicular weight compared to TLR4 mice on HFD (p<0.05; Fig. 2E). The weights of seminal vesicles and epididymis did not show any significant difference between the mice groups. However, the weight of the epididymis and seminal vesicles in the WT and TLR4 mice fed with the HFD diet showed a comparatively higher weight compared to the mice fed with the NCD (Fig. 2F and G).
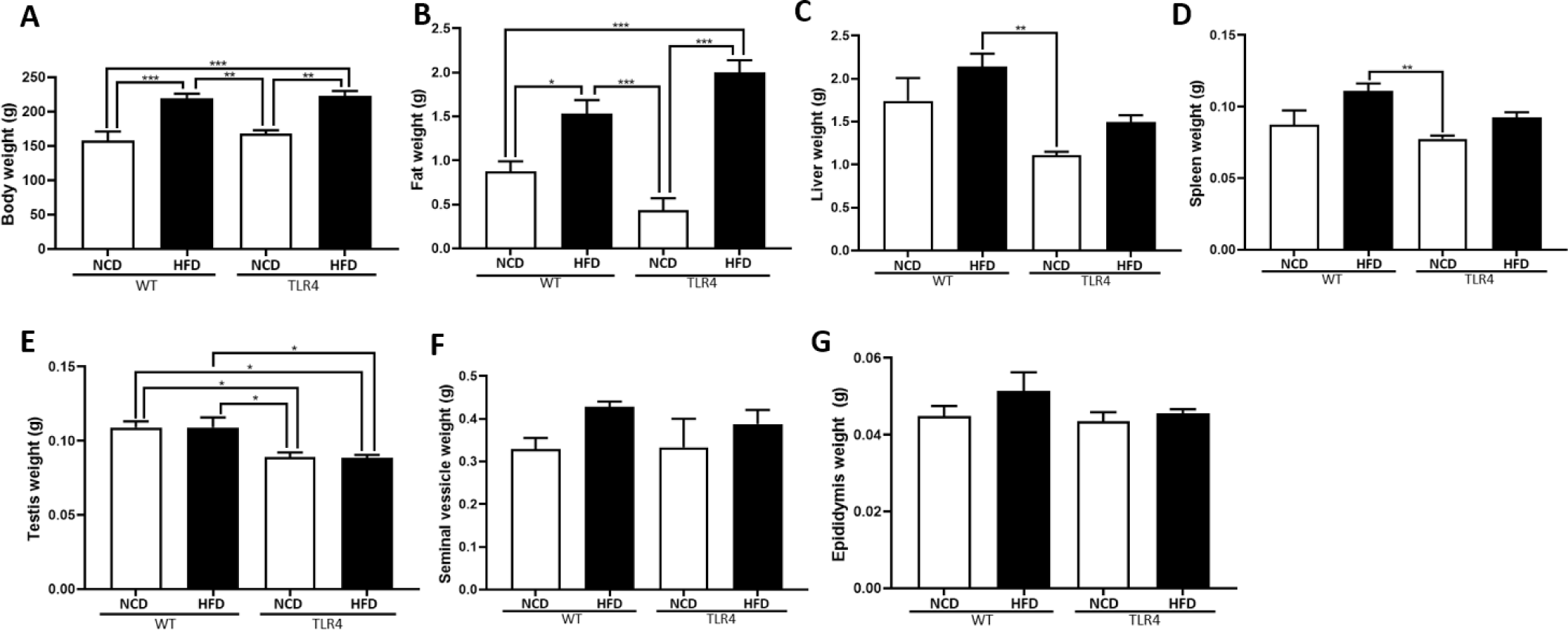
To evaluate the metabolic status of the animals, the serum levels of glucose, triglycerides, cholesterol, and liver enzymes were measured (Fig. 3). WT and TLR4 mice fed a HFD showed increased levels of glucose, triglycerides, and cholesterol (p<0.05, p<0.01, and p<0.001, respectively; Fig. 3A, B, and C). The hepatotoxicity level did not vary between the NCD and HFD-fed TLR4 mice, but a significantly higher GOT level was seen in HFD-WT mice (p<0.001; Fig. 3D). There were no significant differences in the serum levels of GPT among groups (Fig. 3E).
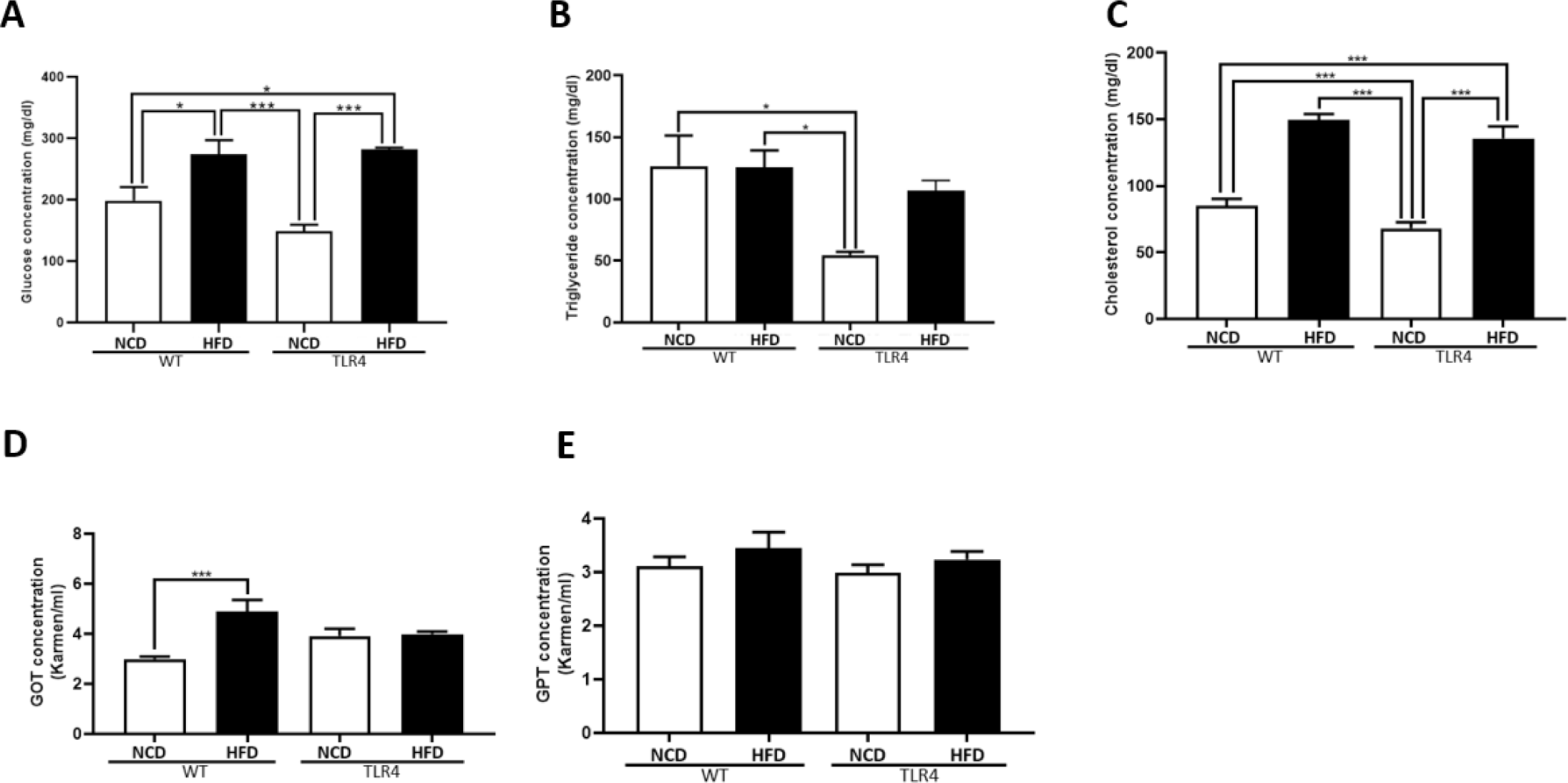
The epididymis was isolated, and the motility and concentration of the epididymal spermatozoa were examined (Fig. 4). Higher sperm motilities were observed in both WT and TLR4 mice fed with a NCD compared to both mice groups fed with an HFD (p<0.01 and p<0.001; Fig. 4A). There was a significantly higher sperm concentration in NCD-WT mice compared to HFD-TLR4 mice (p<0.05; Fig. 4B). The sperm quality was assessed using the proAKAP4 assay kit, which detects the quantity of the proAKAP4 protein in spermatozoa (Fig. 4C). The results showed significantly higher concentrations of proAKAP4 in WT mice fed with a NCD (p<0.05; Fig. 4C).
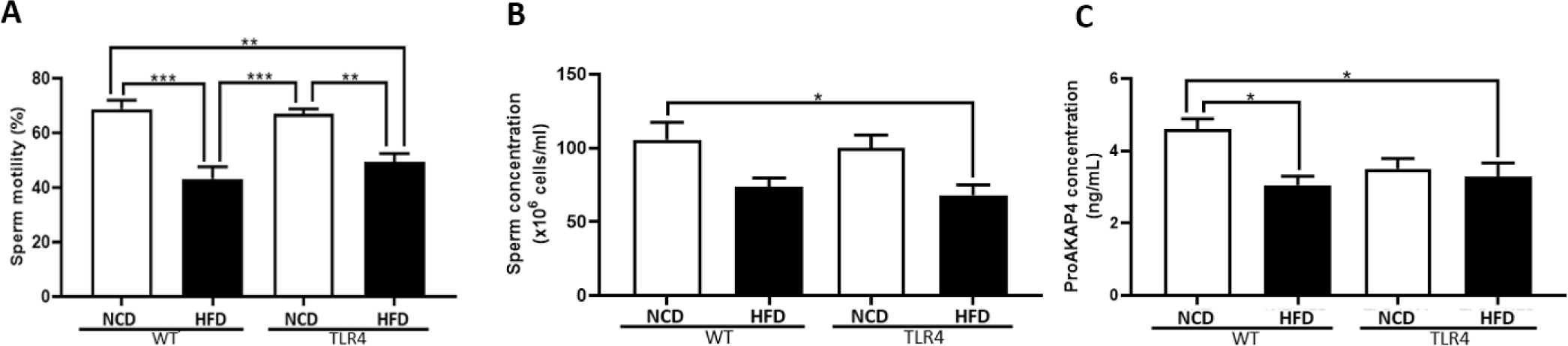
Spermatogenesis in seminiferous tubules was observed in the histological sections of the testis (Fig. 5). A normal arrangement of spermatocytes and spermatids in the basal lamina was observed in the WT mice fed a NCD (Fig. 5A and A’). The TLR4 mice showed relatively loose or narrow seminiferous tubules (Fig. 5C and D) compared to the WT mice (Fig. 5A and B). A decreased or degenerative number of spermatids was observed in the WT mice fed with a HFD (Fig. 5B’). Also, highly defective or degenerative spermatocytes and spermatids were observed in TLR4 mice fed an NCD or HFD (Fig. 5C, C’, D, and D’). However, the sperm morphology compressed out from the center of the tubules seemed to be normal in all the groups (Fig. 5A’–D’).
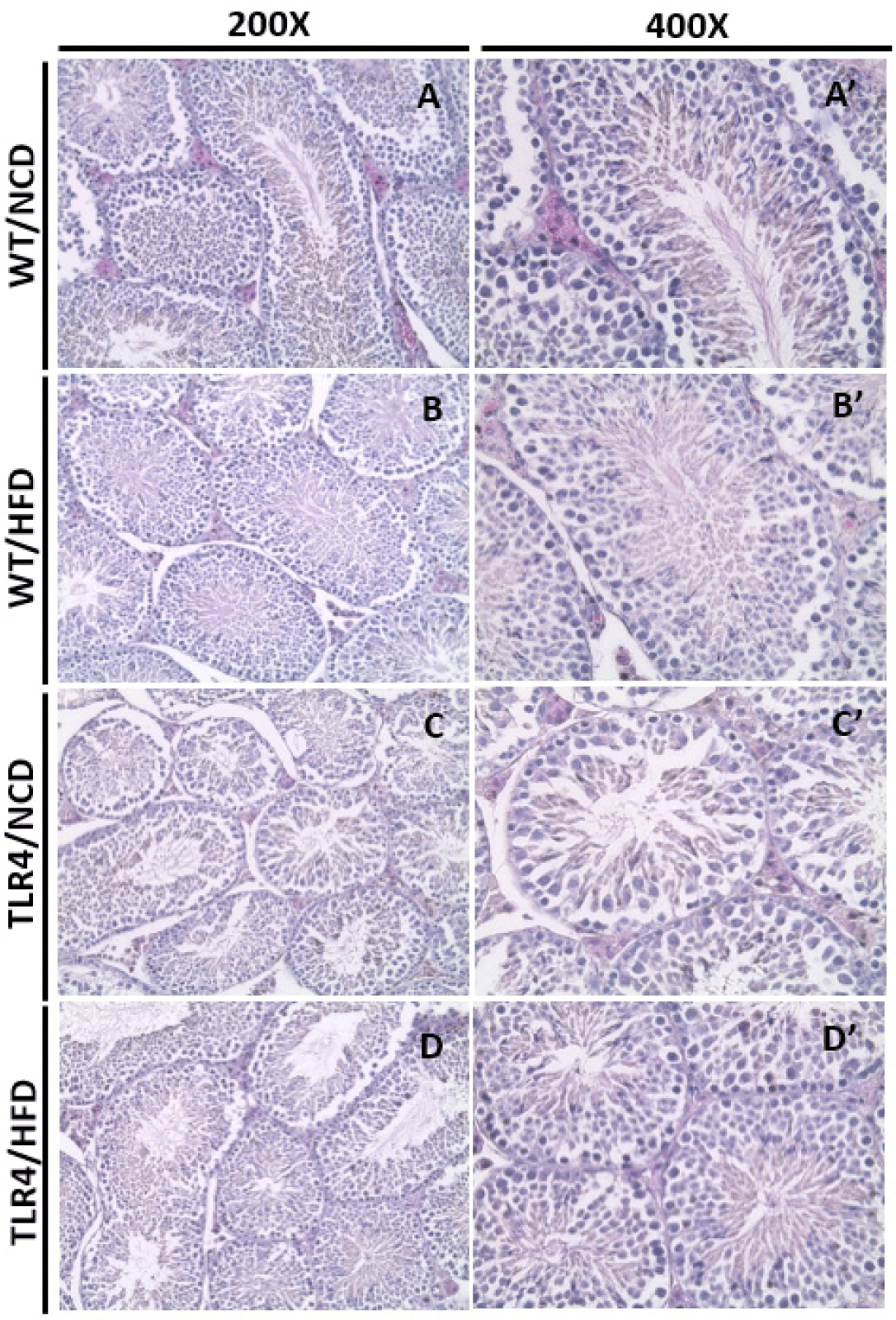
Male mice were randomly selected from each group for mating after being fed a NCD or HFD for 19 weeks (Fig. 1A). WT mice fed a HFD showed a lower pregnancy rate compared to WT mice fed a NCD or TLR4 mice fed a NCD or HFD (p<0.05; Fig. 6A). There was no significant difference in the litter size among groups, but a slightly lower litter size was observed in the WT male mice fed with an HFD (Fig. 6B).
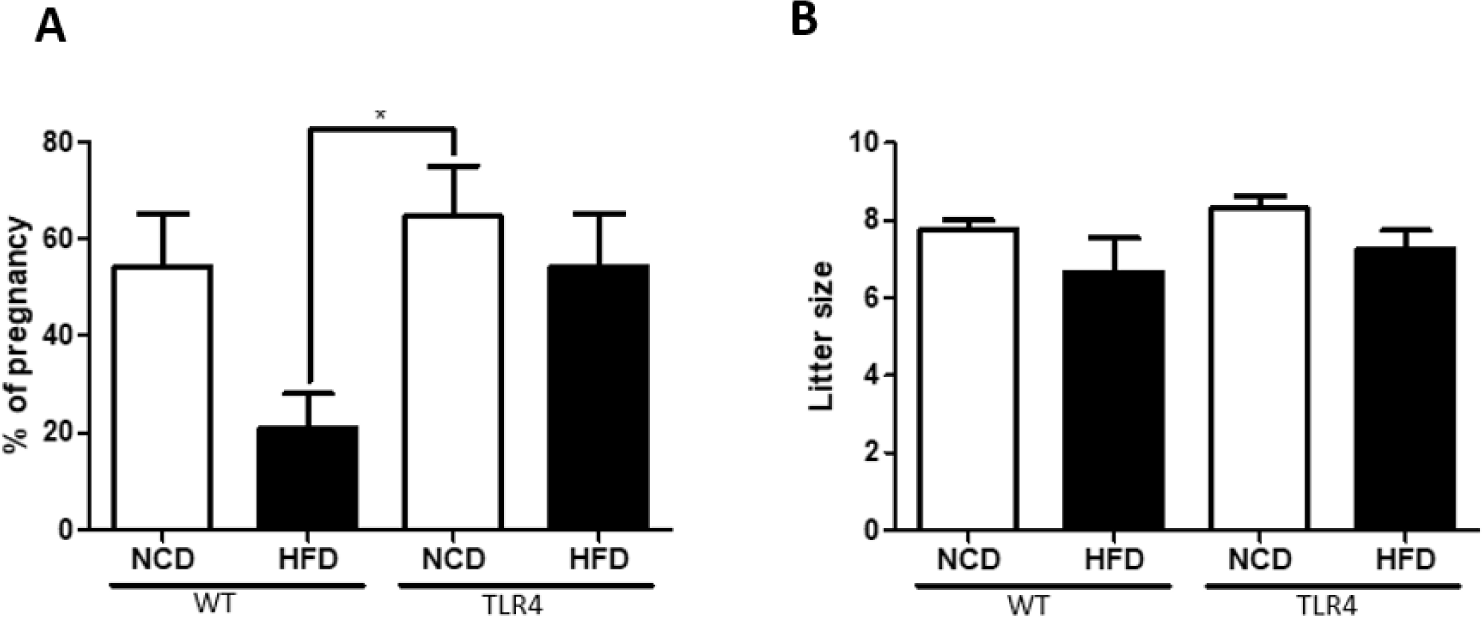
DISCUSSION
The testis is considered a remarkable immune privilege organ in the body as it protects the germ cells from autoimmune attacks. However, inflammatory conditions in the testis, especially those caused by noninfectious conditions are important etiological factors of male infertility [15]. Specific mediators elevated in obese conditions have been identified as triggers that activate the TLR-signaling pathways [16, 17]. A growing body of evidence shows that TLRs play broad roles in testicular function and noninfectious inflammatory responses in the testis which may cause the impairment of male fertility [16, 18]. TLR4 is expressed on the surfaces of macrophage and dendritic cells and activates various inflammatory pathways [19]. The present study was undertaken to determine whether a mutation of TLR4 protects against male infertility in HFD-induced obesity.
In our study, mice were administered with an NCD or HFD for 20 weeks and body and organ weight, serum levels of triglycerides, glucose, cholesterol, and liver enzymes were measured, and the breeding was performed after 19 weeks feeding (Figs. 1, 2, and 3). Regardless of the diet, the body weight gain curves were similar in all the groups (Fig. 1C). Further, high perigonadal fat weight and liver, spleen, testis, seminal vesicle, and epididymis weights were observed in both mice genotypes fed with the HFD compared to those on the NCD (Fig. 2). However when the WT-HFD and the TLR4-HFD groups were compared, the above parameters were lower in TLR4 mice fed with the HFD (Fig. 2). Fat accumulation and a higher liver weight is a manifestation of obesity-related metabolic syndrome [20]. Indeed, while HFD caused an increase in the liver weights in mice of both genotypes, it was comparatively lower in the livers of HFD-fed TLR4 mice. Partially consistent with serum glucose, triglyceride, and cholesterol concentrations and serum hepatotoxicity levels, we observed that HFD feeding in TLR4 mice was less effective compared to the same in WT mice (Fig. 3). Moreover, our findings are similar to the previous findings where they examined the HFD-induced gut microbiota that exacerbates inflammation and obesity in mice via the TLR4 signaling pathway [18].
It has been reported that TLR4 expression increases in obese mice and positively correlates with insulin resistance which results in higher blood glucose levels [21]. The role of TLR4 in obesity was further supported by studies that suggest that TLR4 knockdown protects mice from diet-induced inflammation and insulin resistance [22, 23]. Recent studies reported that the knockdown of TLR4 in the arcuate nucleus protects rats from diet-induced weight gain, glucose intolerance, and peripheral insulin resistance [24]. In agreement with these previous findings, we also observed that HFD feeding in TLR4-deficient mice was less effective on adiposity.
When we considered the reproduction parameters of each mouse group, we observed higher sperm motility in both WT and TLR4 mice fed the NCD diet compared to their corresponding HFD-fed group (Figs. 4 and 5). Mice fed with HFD had significantly higher body weight, which was predominantly a result of an increase in adipose tissue. Increases in levels of serum triglycerides, cholesterol, and glucose were also observed in these mice. The excess adipose tissue was associated with an increase in the local and systemic production of pro-inflammatory adipocytokines which induce the production of reactive oxygen species (ROS) [25]. Furthermore, increased oxidative stress leads to important changes in the adipose tissue, promoting a systemic low-grade inflammatory response with adverse effects throughout the body including the reproductive tract. Therefore, this increase in ROS may have induced lipid peroxidation in spermatozoa which may, in turn, impair sperm motility in the HFD-fed mice groups [26]. The reduction in motility observed in the HFD-fed group in our study is in sync with previously published studies that confirm the negative relationship between sperm concentration, motility, and male obesity [27–29]. In our study, a higher concentration of proAKAP4 which indicates the normal quality of spermatozoa, was seen in sperm isolated from WT mice fed an NCD (Fig. 4C). Spermatozoa with a low concentration of proAKAP4/AKAP4 were categorized as abnormal, immotile, and infertile [14, 30, 31]. In addition, oxidative stress decreases proAKAP4 concentrations in spermatozoa and the proteolysis of proAKAP4 in semen appears as a regulatory sensor of sperm quality and functionality in mammals [30, 31].
In addition, similar to previous studies [32, 33], we observed the deleterious effects of an HFD on the testicular morphological structure. Also, in both WT and TLR4, HFD-induced male obesity resulted in a reduction in fertility in comparison to the NCD-fed mice. However, the effect was more severe in WT mice fed on an HFD. There was no significant difference in the average litter size determined in female mice impregnated by HFD-fed or NCD-fed males (Fig. 6). However, a comparatively lower fertility rate was observed in the HFD-fed male mice compared to the NCD-fed group (Fig. 6A). Thus, overall we have demonstrated a reduction in male fertility in association with diet-induced obesity in mice. Reduced sperm concentration, sperm motility, and quality indicate that obesity may play an important role in the reproductive capabilities of obese mice. However, when we compared the WT-HFD and TLR4-HFD mice groups we observed that male fertility parameters were higher in the TLR4-HFD fed mice compared to the WT-HFD fed mice which indicated that TLR4 mutation in mice affects fertility. It has been reported earlier that various TLRs are expressed in the testicular cells and they play broad roles in testicular function and inflammation [33–35]. TLR4 activation by LPS negatively affects sperm quality implicating ROS production, especially in sperm from infertile patients [36, 37]. The results from our study provide important new insights into how the TLR4 signaling pathway could be responsible for reducing motility and the fertilization potential during HFD-fed obesity. Therefore, fundamental approaches to ameliorate such TLR4-mediated responses may be of potential clinical benefit to infertile patients.
The present study aimed to describe the HFD-related changes in the reproductive capacity of the TLR4 male mice. In general, it has been seen that the spermatozoa of HFD-fed male mice showed decreased motility. HFD-induced obesity has been found to play a significant role in the functions of the male reproductive system. The influence of TLR4 gene-specific functions of the male germ cells during spermatogenesis, especially in WT mice showed a negative impact on HFD rather than in TLR4 mice fed on an HFD. These effects are caused due to obesity and perigonadal fat deposition in the HFD-fed mice from both groups and the decrease in spermatogenesis in male mice. These changes in spermatogenesis lead to an increased proportion of abnormal spermatozoa and a decrease in motility and sperm concentrations in the HFD-fed mice. In conclusion, although HFD alters energy and steroid metabolism in mice, which may lead to male reproductive disorders, fertility was less affected in HFD-fed TLR4 male mice. Therefore, it was found that TLR4 was involved in testicular dysfunction in males due to metabolic imbalance, and additional studies to overcome the decline in fertility through the regulation of related TLRs-inflammatory responses are needed in the future.