INTRODUCTION
Colon cancer is the third leading cause of cancer-associated deaths worldwide, representing the global health burden in recent years [1, 2]. Epidemiological and experimental evidence suggested that lifestyle change, such as diet, is a potent factor in colon cancer occurrence [3–5]. Also, many researchers focused on identifying specific nutrients for the development of primary prevention of colon cancer occurrence. Indeed, some researchers have tried to identify the role of nutrients, including meat, fat, fiber, and vitamins, in colon carcinogenesis [6–8].
Folate is a water-soluble vitamin B9 enriched in dark leafy vegetables, and folic acid is a synthetic compound used as a vitamin supplement [9]. Also, folate supplementation is required for one-carbon metabolism and DNA biosynthesis in proliferating cells [9]. Intracellular folates act as one-carbon moieties and mediate epigenetic modification, such as DNA, RNA, and histone methylation. Low folate levels in plasma are closely associated with reduced colorectal cancer risk and inhibit the progression of preneoplastic and neoplastic lesions [10]. Several prospective cohort studies have investigated the association between folic acid intake and colon cancer occurrence [11, 12]. A recent meta-analysis has shown that colon cancer risk is significantly reduced in subjects with the highest quintile of dietary folic acid intake than the lowest intake [12]. However, another meta-analysis study has presented that folic acid supplementation has no effects on colorectal cancer risk [11]. To identify the effects of folic acid supplementation on colon carcinogenesis, further investigation with a rigorous experimental design is needed.
Reactive oxygen species (ROS), such as hydrogen peroxide, hydroxyl radical, and superoxide anion, are produced during normal energy metabolism [13]. They induce oxidative stress, causing DNA strand breaks, lipid peroxidation, and genetic instability, which are closely associated with colorectal carcinogenesis [14]. Many researchers have presented an oxidative stress-based antitumorigenic strategy with antioxidants and hypoxia adaptation [15]. Several studies have reported the protective effects of dietary folic acid on colon cancer development in animal models [16–18]. However, the role of folic acid-regulated ROS in colon carcinogenesis in experimental animal models is unknown. Therefore, this study aimed to investigate the effects of folic acid supplementation on colon neoplastic change and ROS generation in an azoxymethane (AOM)/dextran sodium sulfate (DSS)-induced colon cancer mouse model.
MATERIALS AND METHODS
AOM and folic acid were obtained from Sigma-Aldrich (St. Louis, MO, USA). DSS (molecular weight 36,000–50,000) was obtained from MP Biomedical (Santa Ana, CA, USA).
Four-week-old male institute for cancer research (ICR) mice were purchased from Samtako Bio Korea (Osan, Korea) and housed in an isolating mouse cage (five mice per cage). The temperature and humidity were set at 20 ± 2°C and 50 ± 20%, respectively. Purified diet AIN-76A was purchased from Central Laboratory Animal (Seoul, Korea). During the experimental periods, all diets and litter were used after sterilization. The entire animal experiment was performed in compliance with the Guide for Care and Use of Laboratory Animals of Chungbuk National University (Institutional Animal Care and Use Committee [IACUC] approval no. CBNUA-1139-18-01). Body weight and food consumption were recorded weekly.
After acclimation to the cage for 1 week, 30 mice (5 weeks old) were divided into control and experimental groups. AIN-76A diet and distilled water were supplied to mice. Folic acid (0.03%) was supplemented via drinking water (50 mL/week/mouse) to the experimental group. To induce the formation of preneoplastic lesions in the colon, AOM (10 mg/kg body weight) was subcutaneously injected thrice at 0, 1, and 2 weeks of the experimental period. At the third week of the experimental period, distilled water with 2% DSS was provided for 7 days. The total experimental period was 6 weeks. At the termination of the study, mice were sacrificed by cervical dislocation to examine the colon. After mice were sacrificed, entire large intestine samples were collected. Feces were obtained in the cage before sacrifice. Large intestine samples were washed with saline and fixed in 10% neutral buffered formalin. The detailed experimental design is described in Fig. 1.
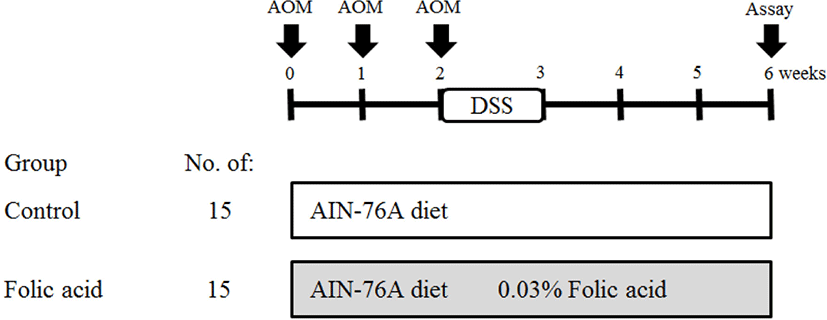
Colons were collected and rinsed with 0.9% NaCl solution. Colons were then fixed flat in 10% neutral phosphate-buffered formalin. Fixed colons were stained with 0.2% methylene blue for 2 s. Colon samples were placed on the mucosal surfaces of a slide for examination under a light microscope. The total numbers of ACF and AC in each focus were counted under a microscope (× 40 and × 100; Fig. 2).
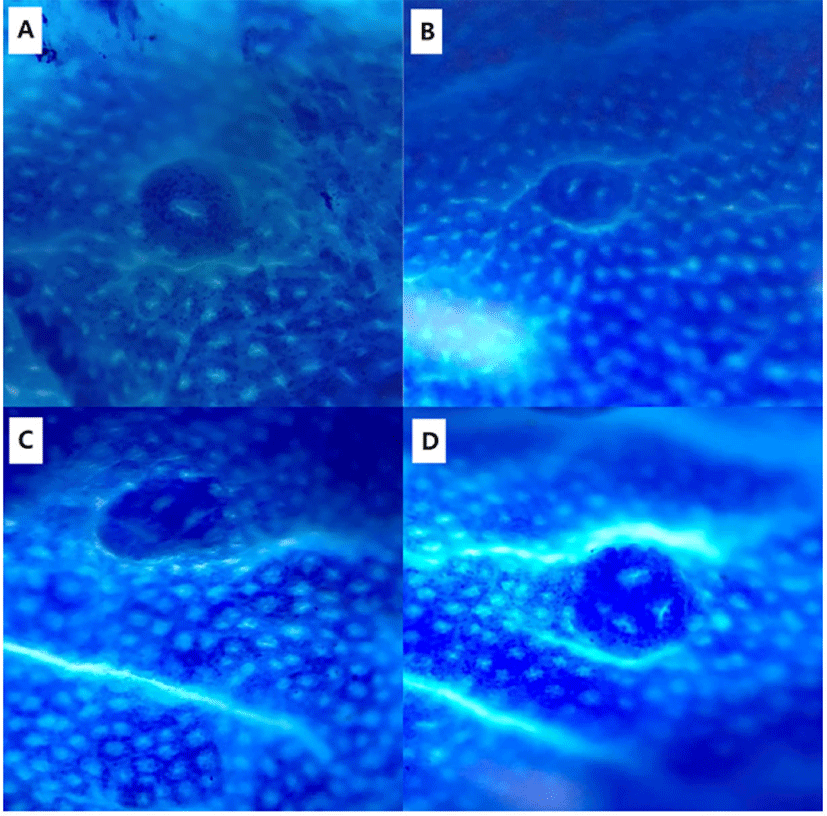
Tissue samples were fixed in 10% neutral buffered formalin. Fixed tissue was paraffin-embedded and then cut to multiple 4 μm sections. Sliced samples were stained with hematoxylin and eosin (H & E) for histopathological examination under a light microscope (Olympus, Tokyo, Japan).
The dry feces of five mice were diluted with distilled water and then incubated at 37°C for 1 h. The samples were centrifuged for 15 min at 15,000 × g, and the supernatant was collected. Malondialdehyde (MDA) levels were detected using the TBARS Assay Kit (Cayman Chemical, Ann Arbor, MI, USA). The assay was carried out according to the supplier’s instructions.
All statistical analyses were performed using GraphPad Prism 5 software (GraphPad, San Diego, CA, USA). Comparisons of two groups were performed using Student’s t-test. Data were expressed as the mean ± S.D. Significant differences were determined at the level of p<0.05 or p<0.01.
RESULTS
To identify the effects of folic acid supplementation on colon carcinogenesis of the AOM/DSS mouse model, the body weight of an AOM/DSS mouse model was investigated weekly. All mice grew at a similar rate throughout the study. During the entire experiment, the control group were heavier than the folic acid supplementation group (Fig. 3). At the end of the experiment, the final weight of the two groups was not significantly different (Fig. 3). Also, H & E staining for colon epithelium was performed to identify the effects of folic acid supplementation on histological changes in an AOM/DSS mouse model. As shown in Fig. 4, the folic acid supplementation group revealed ameliorated hyperplastic change in colonic mucosa compared to the control group. Concerning that ACF and AC formations are considered the earliest morphological changes during colon carcinogenesis, the modulatory effects of folic acid on ACF and AC formation in colon tissue were checked. The total numbers of ACF and AC lesions in colon tissue of an AOM/DSS mouse model were measured. As shown in Figs. 5 and 6, the total number of ACF in the folic acid supplementation group (5.7 ACFs) decreased compared to controls (9.3 ACFs). Also, the total number of AC in the folic acid supplementation group (7.5 ACs) decreased compared to the control group (12.4 ACs). These data indicated folic acid supplementation suppresses hyperplastic alteration in colon tissue of an AOM/DSS mouse model.
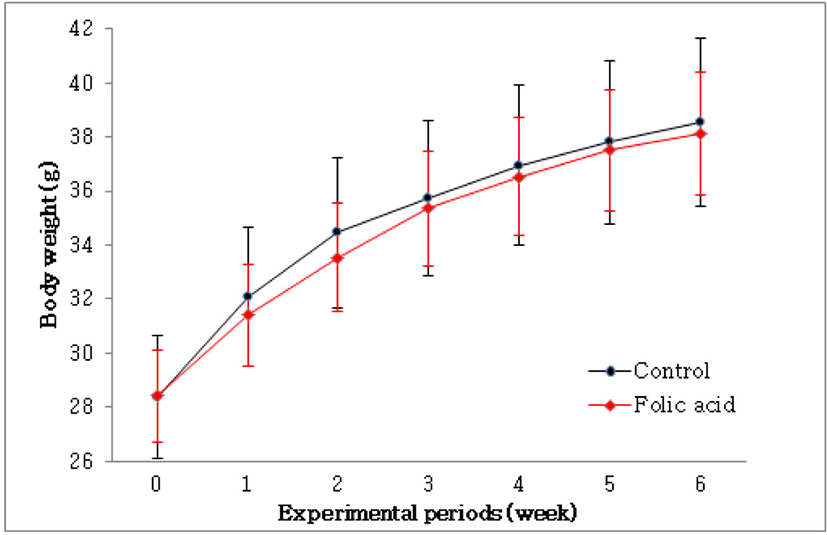
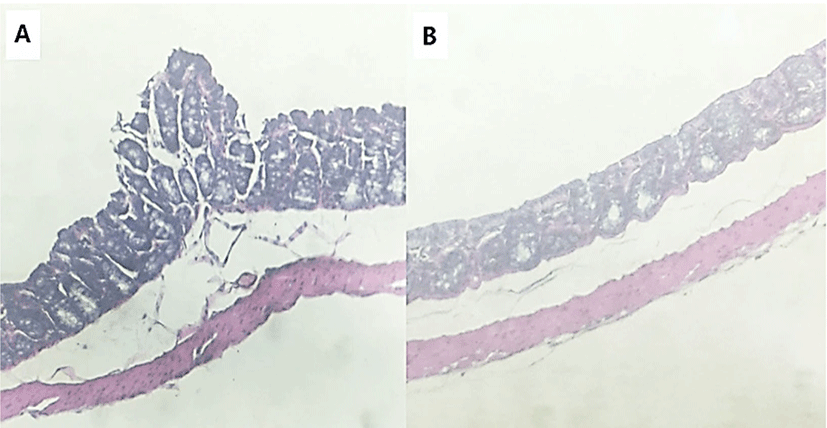
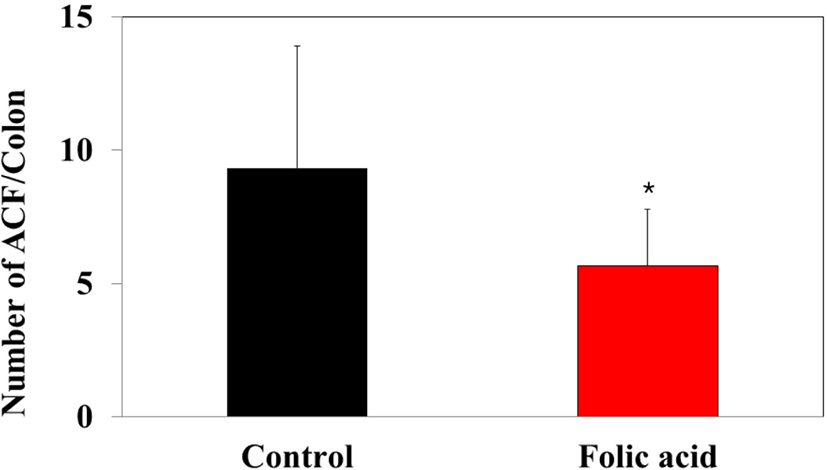
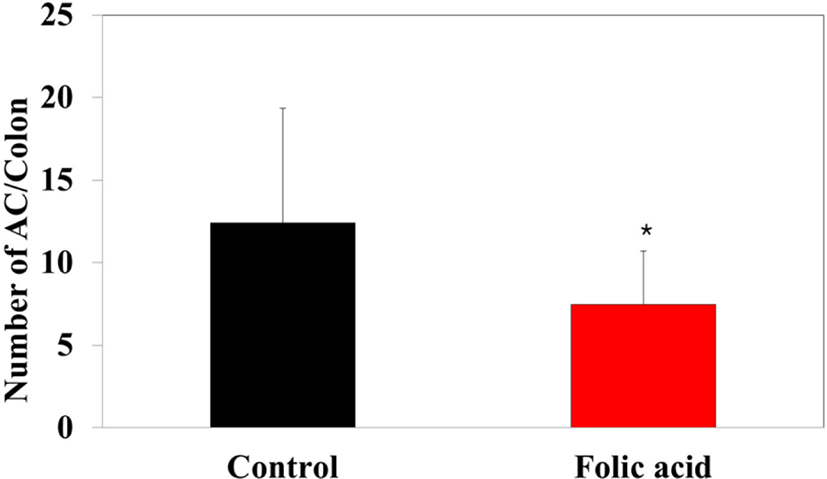
MDA concentrations in dry feces have been considered markers for oxidative stress-stimulated lipid peroxidation in the colon mucosa. To investigate the regulatory effects of folic acid supplementation on oxidative stress of an AOM/DSS mouse model, a TBARS assay was performed with dry feces samples. The TBARS concentration in the folic acid supplementation group (8.3 μM) significantly decreased compared to controls (16.6 μM; Fig. 7). This observation revealed the antioxidative effects of folic acid supplementation on an AOM/DSS mouse model, suggesting the possibility of close relationships between folic acid antioxidation and colon carcinogenesis.
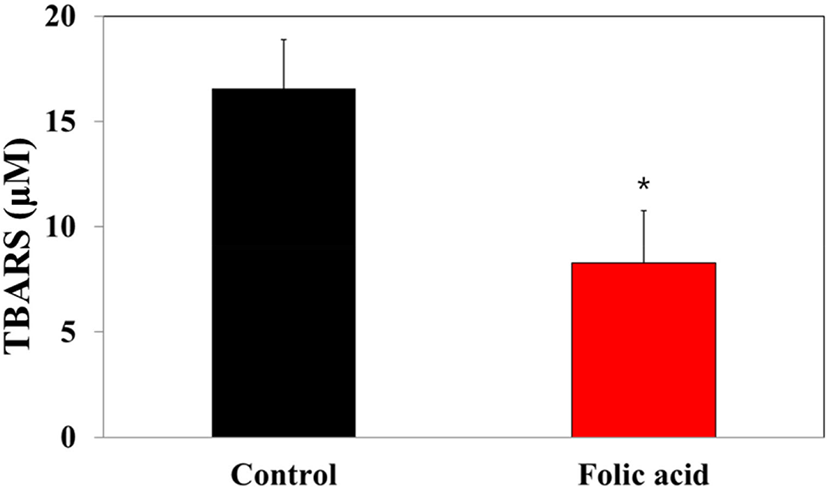
DISCUSSION
This study demonstrated that folic acid supplementation suppresses AOM/DSS-induced colon carcinogenesis and ROS accumulation in an in vivo model. AOM is a very potent carcinogen that induces colon cancer with a high incidence in experimental animals [19, 20]. AOM-induced methyl diazonium ion is a highly reactive electrophile that increases oxidative stress in the liver and colon tissue [21]. Also, AOM induces ACFs that are incipient lesions in colon cancer development [22]. ACF is identified by microscopy on methylene blue-stained colon mucosa and characterized by enlarged and risen dark crypts [23]. Therefore, ACFs are used as colon cancer biomarkers [24]. This study also found histological alterations, including AC and ACF formations with hyperplastic change, in an AOM/DSS-induced colon carcinogenic mouse model.
Although some meta-analysis reports suggested that folate supplementation does not affect colorectal cancer risk, many researchers reported the therapeutic effects of folic acid on various types of cancers, including colorectal cancer [10, 11, 25, 26]. Also, targeting the folic acid receptor α has been considered a promising targeted therapeutic strategy in oncology [27]. Consistent with these findings, the protective effects of folic acid on an AOM/DSS mouse model are presented.
Present study showed that folic acid supplementation significantly alleviated the hemin-induced ROS generation in colon carcinogenesis mouse model. Elevated ROS levels have been well documented in various types of cancers [28–30]. Furthermore, oxidative stress-induced DNA damage causes colorectal carcinogenesis by genetic mutation [13]. Previous reports suggested that the protective effects of folic acid are related to the methionine cycle. In the methionine cycle, homocysteine derived from methionine contributes to cysteine formation, which is essential for the formation of the antioxidant molecule glutathione (GSH) [31]. Folate-regulated GSH expression has a critical role in redox homeostasis [31]. Consistent with these findings, many reports showed evidence supporting the antioxidative effects of folic acid supplementation. In an AOM-induced colon carcinogenesis rat model, folate and vitamin B12 supplementation alleviates AOM-stimulated GSH depletion and protein oxidation and DNA oxidative damage [32]. One report showed that a folate-deficient tumor microenvironment increased ROS and metastasis but decreased proliferation in human hepatocellular carcinoma [26]. Also, tert-butyl hydroperoxide-induced oxidative stress decreased folic acid uptake by suppressing folate transporter mRNA, which plays a key role in the proliferation and viability of colorectal cancer cells [33]. These and previous findings indicated that folic acid-suppressed oxidative stress plays a key role in preventing ROS-induced colon carcinogenesis through the regulation of genetic mutation and cancer pathogenesis.
In conclusion, the results have shown that folic acid prevents ROS accumulation and colon carcinogenesis, although most antitumorigenic therapeutic strategies employ oxidative stress to disturb the cellular redox state and cancer cell death [13]. Considering that colon carcinogenesis in an AOM/DSS mouse model is related to excessive oxidative stress, this study suggests folic acid supplementation therapy as a preventive strategy for patients with a high risk of colon cancer.