Introduction
Expeditious development in the fields of agriculture, medicine and manufacturing industries increases the environmental pollution due to anthropogenic activities [1]. Heavy metals are especially responsible for increasing the pollution due to improper waste handling, which ultimately results in undesirable consequences on the human health [2, 3]. Cadmium (Cd) is a naturally inhabited heavy metal, increasing in abundance due to anthropogenic activities of mankind, and subsequent increase in human exposure [4]. Humans can be exposed to Cd through the long-term consumption of contaminated food and water, and inhalation of the smoke [5]. Cd has the ability to replace calcium in minerals because of its similar ionic radius, identical charge, and similar chemical behavior [6]. Therefore, it can easily be ingested by the human body and accumulate in certain organs [7]. Cd is a known neurotoxin and targets organs such as the brain, lungs, liver and kidneys [5, 8, 9]. Moreover, it causes renal and hepatic dysfunction, testicular damage, osteomalacia, pulmonary edema, and damages the adrenals [10]. It is also a proven carcinogen, and is the causative agent of numerous lung, breast, pancreas, urinary bladder and nasopharyngeal malignancies [11].
Thus, there is an urgent necessity to evaluate the toxic effects of cadmium in the living body, and finding a suitable animal model is a critical factor to achieve this. Fresh water planarians are known to be free-living flatworms, having great anatomical suitability for toxicity studies [12]. They have the ability for both sexual and asexual reproduction as well as regeneration, and a simple lifecycle [12, 13]. They also encompass a well-developed central nervous system which is similar to humans [14]. Moreover, planarians are easy to manage, requiring less space, can easily be observed by the naked eye, and are comparatively inexpensive than other laboratory animal species [15, 16]. Most importantly, compared to other alternative toxic models like nematodes and zebrafish, planarians are highly sensitive to environmental chemicals [17, 18], especially their behaviors, regeneration ability and brain structure [19]. Considering all the above factors, the present study was performed to evaluate Cd toxicity in planarian (Dugesia japonica), and to determine the suitability of planarian as an animal model for toxicity studies.
Materials and Methods
Approximately 1.0 cm long planarians (Dugesia japonica) were used as the animal model in this study. Planarians were acclimated in freshwater at 18°C–22°C, and fed finely homogenized beef liver twice a week. One week prior to the experiment, the planarians were starved to create a uniform metabolic state. Cadmium sulfate (3CdSO4 × 8H2O; Cds) was purchased from Sigma-Aldrich (Seoul, Korea). Chemicals were dissolved in distilled water (DW) before experimental application.
Motility was measured by evaluating swimming ability of the planarian in its natural state. Briefly, petri dishes (p-dish) filled with treatment solutions were placed on a graph paper, which was divided into a grid of 0.5 cm intervals. Motility was evaluated by counting the number of grid lines that the planarians passed through. Simultaneously, planarian seizure-like behaviors were also observed, and the actions were classified into four categories: c-like, head-bop, snake-like, and screw-like [20, 21]. Planarians were incubated in 10 ml experimental solutions containing 0.1–25 mg/L cadmium sulfate (Cds) for 1–5 hrs, after which they were introduced into the respective petri dish for each treatment solution separately. Motility and seizure-like patterns were subsequently measured (number of grid lines crossed or re-crossed by each planarian) within 5 min.
Regeneration assay was conducted with a minimum number of planarians (n = 10) in each treatment solution. Planarians were paralyzed by chilling on ice, and the stretched bodies were cut by using an ethanol-sterilized blade. The head region on the auricular grooves was cut, and rest of the body was incubated in water until emergence of eye extrusion. Each amputated fragment was transferred to a 12-well plate filled with the treatment solutions, and maintained in a dark environment at 18°C–22°C. The imaging process of the regeneration fragments was conducted for 14 days, using a stereo-microscope (S8APO, Leica, Wetzlar, Germany).
Immunofluorescence staining was conducted with slight modification [22]. Briefly, regenerating planarians were treated with 2% HCl diluted in DW for 5 min to remove excess mucus, after which they were fixed in Carnoy’s solution (ethanol : chloroform : acetic acid = 6 : 3 : 1) for 3 hrs at RT. Subsequently, samples were rinsed with 100% methanol and bleached overnight at RT in 6% H2O2 diluted in methanol under a light emitting diode (LED) lamp. Bleached samples were rehydrated through a graded methanol series (75%, 50%, and 25%) diluted with phosphate buffer saline (PBS), 15 min in each solution. Samples were then rinsed in PBS containing 0.3% Triton X-100 (PBST), and blocked in PBST containing 0.25% BSA (PBSTB) for 2 hrs, followed by incubation with rabbit polyclonal anti-arrestin antibody (LAgen Laboratories, 1:5,000 dilution) in PBSTB, overnight at 4°C. Probed samples were washed 3 times with PBSTB for 2 hrs, and subsequently incubated with goat rabbit anti- Alex488 IgG antibody in PBSTB (Invitrogen, 1:2,000 dilution) and 0.5 µg/mL 4’6’-diamidino-2-phenylindole (DAPI; DNA), overnight at 4°C in the dark. After incubation, samples were washed with PBST. Finally, the samples were mounted on a slide and observed using a fluorescence microscope (Nikon Eclipse Ci, Nikon, Seoul, Korea).
Results
Planarians were incubated in water containing varying concentrations of Cds for 1–5 hrs (Fig. 1). Compared to the control (without Cds), motility was significantly and dose-dependently decreased in Cd concentrations at varying incubation times (p<0.05 & p<0.001; Fig. 1A–C). After incubation, the lethal concentration (3–5 hrs LC50) of Cds was estimated at 5 mg/L (Fig. 1D). Also, seizure- like behaviors were observed in planarians exposed to Cds (Fig. 2). Higher numbers of c-like behavior were observed in planarian exposed to varying concentrations of Cds for 1–3 hr incubation, compared to the control without Cds (p<0.05 & p<0.01; Fig. 2A). Behavior patterns, including head bop, snake-like and screw- like movements, were observed in planarian exposed to Cds for 1–3 hrs incubation, whereas either no seizure- like behaviors were observed or most of them died after 5 hrs incubation in the presence of Cds (Fig. 2B–D). These results indicate that normal behavioral patterns are altered in planarians incubated in the presence of Cds.
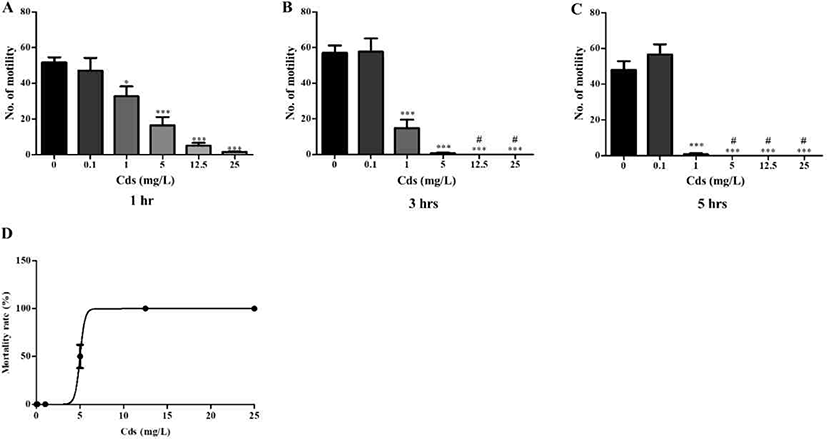
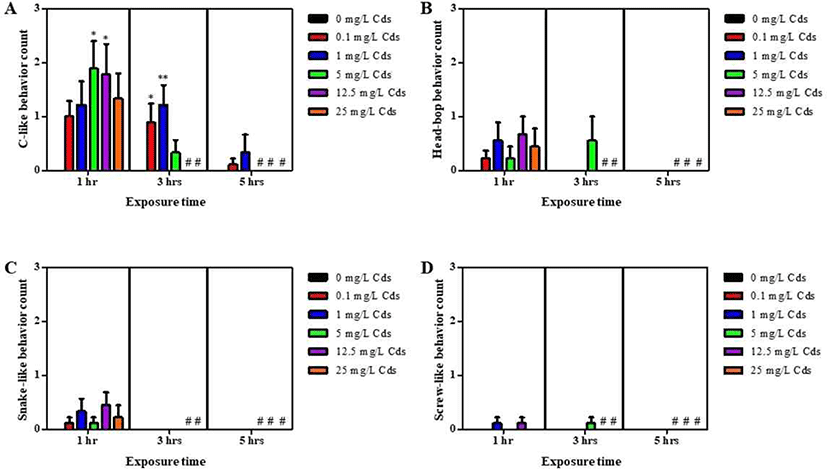
In order to observe eye regeneration from amputated fragments, the head region on the auricular grooves was cut, and rest of the body was incubated in water until emergence of eye extrusion. The degree of regeneration was calculated by indicating a score of 0, 0.5, and 1, depending on burst, regeneration and completion of regeneration, respectively, as shown in Fig. 3A.
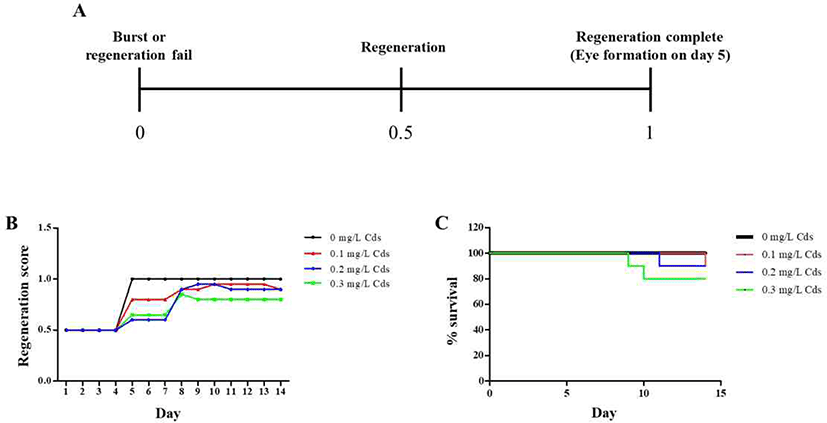
On day 5, the eye spot was observed in planarians incubated in water without Cds; however, planarians exposed to Cds showed either no formation or delayed formation of eye spots (Fig. 3B). This pattern lasted in planarians incubated in the presence of Cds until the 8th day, after which the eye spots appeared. In particular, planarians cultured at concentrations above 0.3 mg/L showed low eye formation score for 14 days (Fig. 3B). Moreover, planarians incubated in the absence of Cds showed 100% survival for 14 days, but the lifespans of planarians exposed to Cds were decreased, and survival was less than 80% in planarians incubated in the presence of 0.3 mg/L Cds (Fig. 3C).
As presented in Fig. 4, eye formation was clearly observed in water without Cds or with 0.1 mg/L Cds on day 5 (Fig. 4A and B), whereas 0.2 mg/L Cds exposed planarian was unable to form eye spots on day 5, but finally formed eye spots on day 8 (Fig. 4C). Planarians in all treatment groups completed eye formation on day 14; thus, higher concentrations of Cds induced delayed eye regeneration.
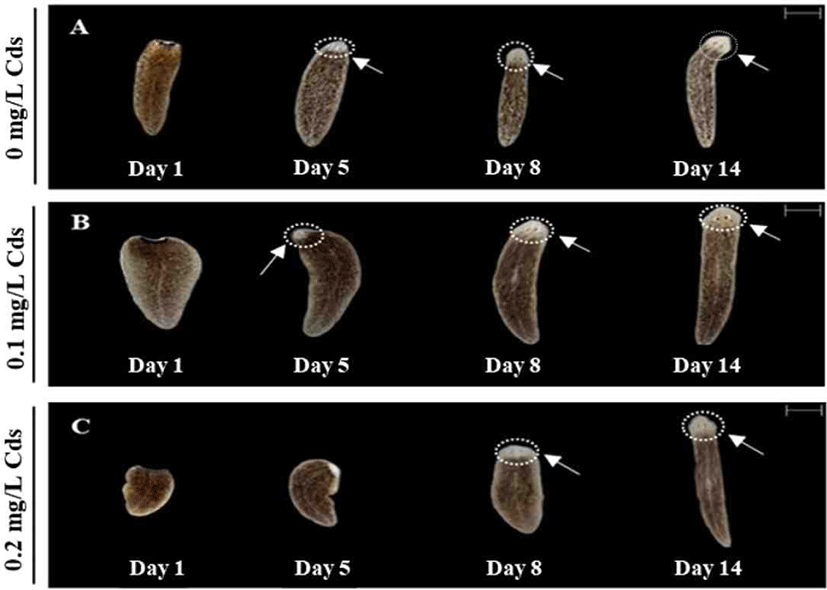
Optic nerves were observed by immunohistochemistry using anti-arrestin antibody (Fig. 5). Amputated fragments in each treatment were cultured for 14 days (Fig. 5A, D & G), and eye spots appeared in planarian cultured without or with 0.1 mg/L Cds on day 5 (Fig. 5B & E). Delayed eye formation was observed in planarian cultured in 0.2 mg/L Cds on day 5 (Fig. 5H). Eye spots (blue; DNA) and nerves (green; arrestin) were observed in planarian incubated without or with 0.1 mg/L (Fig. 5C & F), but there was no nerve formation in planarian exposed to 0.2 mg/L Cds (Fig. 5I).
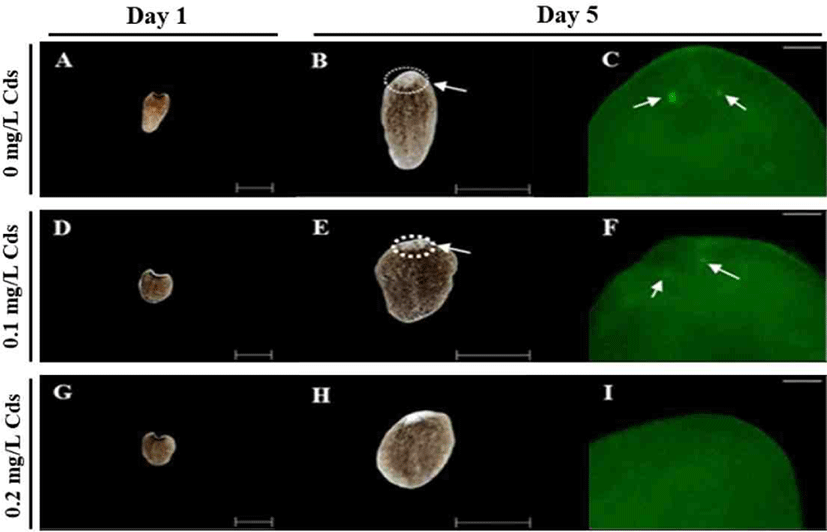
Discussion
Rapid development in the economic sector has resulted in numerous natural and man-made toxins being discharged into the natural environment [1]. Heavy metal pollution is increasing due to the industrialization process and anthropogenic activities of humans [1, 23, 24]. Among the heavy metals, Cd is one of the most ejected metals in a wide range of industries [25]. Human exposure to Cd occurs through the occupational environment or the long-term in-contact with common goods available in their immediate environment, such as Cd-containing common products like batteries and artist paints [26].
Cd is toxic at low concentrations and has the ability to act as a carcinogen. It is mainly absorbed through the respiratory tract, while relatively smaller amounts are absorbed through the gastro-intestinal tract and the skin [27]. In the present study, planarians exhibit a dose-dependent decline in motility and increase in seizure-like behaviors (Fig. 1 & 2). Moreover, their ability to regenerate was reduced when exposed to Cd (Fig. 3–5). Exposing fresh water planarians to high concentrations of Cd also resulted in altered neurobehaviors and changes in the morphology (shape and body elongation). These results are similar to a previous study, which reported that exposing planarians to Cd causes disturbances to their neurotransmission system [28].
Cd accumulation in the body induces cardiovascular diseases, kidney disease, metabolic syndrome, and cancer [29]. Calcium is an essential element for biochemical reactions in the body. Due to similar chemical characteristics (ionic radius, charge and behavioral pattern) [5], Cd competes with calcium ions in the body, and causes oxidative stress by inducing reactive oxygen species (ROSs) [30]. Moreover, when Cd enters the body, it induces increased levels of ROSs, which ultimately induces DNA damage and interferes with the DNA repair mechanism [30]. Cd exerts its toxic effect by blocking the electron transfer chain of mitochondria, where Cd ions impair the electron flow [5]. Numerous experiments related to heavy metal toxicity have been studied in invertebrate animals. The long-term exposure or high dose intake of trace metals alters the antioxidant activity of the body by inhibiting the antioxidant enzymes, resulting in decreased antioxidant capacity in the body [31, 32], which subsequently leads to accumulation of ROS, causing tissue damage and cell apoptosis [33], inhibition in the activity of total superoxide dismutase (SOD), and an increased malonaldehyde (MDA) content [34]. Several research articles on crustaceans have proved that Cd exposure leads to an increase in the MDA content [33–35]. Although examination of the antioxidant activity was excluded in this study, it is presumed that Cd is involved in antioxidant functions and delayed cell regeneration. These experiments will be done in subsequent studies.
Taken together, results obtained in this study indicate that behaviors of planarians are altered after exposure to Cds, along with delayed eye formation. This study therefore presents the risks of direct toxicity and heavy metal exposure that are likely to occur when exposed to Cd. This study further validates the suitability of planarians as an alternative animal model in toxicity studies.