Introduction
Carcass grading is the term used in carcass classification for the purpose of trading encouragement and customer satisfaction [1]. Carcass grade mainly depends on the marbling score, rather than meat color, fat color, firmness, or texture in Hanwoo. Hanwoo generally has intramuscular fat, and 1++ grade Hanwoo are particularly high in fat and low in moisture. Comparatively, Hanwoo possess low cholesterol levels and high levels of fatty acids including monounsaturated fatty acids (MUFA) compared to other cattle [2, 3]. Moreover, high grade Hanwoo carcasses have high levels of MUFA whereas low grade Hanwoo carcasses present high levels of saturated fatty acids (SFA) [4].
Normally, the majority of body fat consists of triglycerides (TG). Following de novo lipogenesis (DNL), hepatic triglycerides (TGs) are synthesized by combining three fatty acids with one glycerol molecule. During DNL, sterol regulatory element binding protein 1 (Srebp-1) is a key contributor that regulates transcriptional levels of several genes including acetyl CoA carboxylase (Acc), fatty acid synthase (Fasn), stearoyl CoA desaturase (Scd1), and glycerol-3-phosphate acyltransferase 1 mitochondrial (Gpam) [5]. In fatty acid synthesis, some of the polyunsaturated fatty acids (PUFA) are converted into MUFA by Scd1 in the endoplasmic reticulum (ER) of liver cells. Moreover, TG and MUFA synthesis is promoted by Srebp-1 [6].
Recently, whole genome sequencing of Hanwoo has been achieved [7]. Moreover, there have been several studies focusing on the expression of genes associated with marbling [8, 9]. Especially, fat-associated genes have been screened in intramuscular fat, and the level of Gpam is significantly positively correlated with carcass grade in Hanwoo [10]. While lipid metabolic genes seem to be important, another feature of high grade carcasses is the induction of glycolysis after tenderization processes such as electrical stimulation [11].
Based on a previous report which investigated the metabolic role of Pgrmc1 under a high fat diet [12], we expected that genetic or epigenetic factors related to Pgrmc1 could influence the carcass grade of cattle. Pgrmc1 is a novel cellular membrane receptor associated with cholesterol synthesis and steroidogenesis. More importantly, Pgrmc1 interacts with the Insig-1/Scap/Srebp-1 complex and its loss triggers high levels of mature SREBP-1 protein. As high grade Hanwoo carcasses feature high levels of MUFA and lipid synthesis, we speculated that Pgrmc1 might be closely related to higher carcass grades in Hanwoo. We were able to observe the expression level of Pgrmc1 in the livers and its association with lipids, lipid metabolism genes, and glycolytic genes.
Materials and Methods
Livers of 52 male Hanwoo were kindly provided by Institute of Health and Environment, Daejeon Metropolitan City. 1++ (6 cows), 1+ (13 cows), 1 (25 cows), and 2 (8 cows) were classified into group 1++or 1+, group 1, and group 2 carcass grade.
Total RNA of Hanwoo liver and Hep3B cells were extracted with the TRIzol® Reagent (Thermo Fisher Scientific, MA, USA), Chloroform (C2432, Sigma-Aldrich, Gillingham, UK), Isopropanol (1.09634.1011, Merck, Darmstadt, Germany), and DEPC (E174, Amresco, West Chester, PA, USA)-treated water. cDNA was obtained by reverse transcription with 1–1.5 μg of total RNA and Reverse transcriptase kit (SG-cDNAS100, Smartgene, UK). Quantitative PCR (Real-time PCR) was performed with specific primers (Table 1) and Excel Taq Q-PCR Master Mix (SG-SYBR-500, Smartgene, Ljubljana, Slovenia). Stratagene Mx3000P (Agilent Technologies, Santa Clara, CA, USA) equipped with a 96-well optical reaction plate was used.
Beef marbling score | Strong positive | Slight positive | Negative | Positive ratio (%) |
---|---|---|---|---|
1++/1+ | 1 | 3 | 15 | 21.1 |
1/2 | 1 | 2 | 30 | 9.1 |
For Oil-Red-O, frozen tissue were cut by 7 μm after embedded with optimal cutting temperature (OCT) compound and attached to silane coated slide. After drying 10 mins in RT, slides were fixed with formalin for 20 mins and washed with running tap water for 10 mins. Slides were proceeded to rinse step with 60% isopropanol and stained with Oil Red O working solution (3 g/L) for 15 mins. After washing with 60% isopropanol, slides were stained with hematoxylin for 30 secs and rinsed with distilled water. Region of interest was observed by light microscope after mounted in aqueous mountant.
Cell culture reagents were obtained from Welgene (Gyeongsan, Korea). Hep3B cells were obtained from Korean Cell Line Bank (KCLB, 88064). Hep3B cells were incubated in Dulbecco’s Modified Eagle Medium (DMEM) (Welgene) containing 5% (vol/vol) foetal bovine serum, penicillin (100 U/mol) and streptomycin (100 μg/mL).
siRNA transfection was performed with lipofectamine 2000 (11668-027, Thermofisher, Waltham, MA, USA) according to the manufacturer’s protocol. Negative control siRNA and PGRMC1 siRNA #1 and #2 were purchased from Bioneer (Daejoen, Korea). The sense sequences of PGRMC1 siRNA #1 and #2 were 5’-CAGUACAGUCG CUAGUCAA-3’ and 5’-CAGUUCACUUUCAAGUAUCA-U-3’.
Results
To investigate whether fatty livers occur in high carcass grades, we performed Oil-Red-O staining to evaluate TG status. As a result, we observed fatty livers and classified them as strongly positive, slightly positive, or negative. Representative images for strongly positive, slightly positive, and negative are shown in Fig. 1. Carcasses grade 1++/1+ comprised 21.1% of Oil-Red-O positive livers and carcasses grade 1/2 carcasses constituted 9.1% of Oil-Red-O positive livers (Table 1). As the percentage of Oil-Red-O positive livers increased with carcass grade, we speculated that fatty liver is induced in high carcass grades.
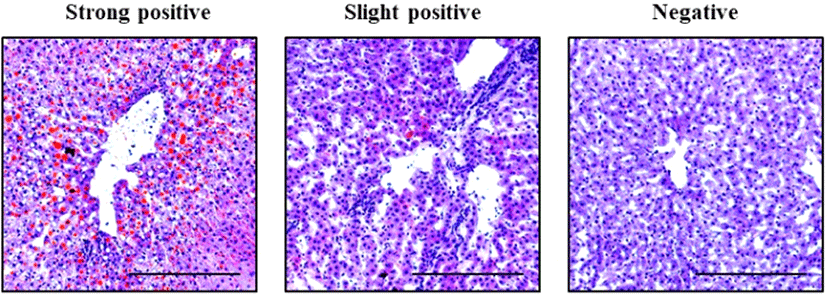
As fatty liver is induced by DNL in a series of steps, we evaluated the mRNA levels of genes associated with DNL. Unexpectedly, the mRNA levels of Srebp-1 were not significantly different between groups (Fig. 2A). Nevertheless, as SREBP-1 protein regulates the transcription of several enzymes, we continue to monitor its mRNA levels. As a result, mRNA levels of Acc (4.31-fold) and Fasn (2.09-fold) were significantly higher (p<0.05) in carcass grade 1++/1+ compared to levels in the livers from grade 1/2 carcasses (Fig. 2B and 2C), suggesting that fatty acid synthesis was induced. Moreover, Scd1 mRNA levels were significantly higher (p<0.05, 2.77-fold) in grade 1++/1+ carcass than in grade 1 carcass (Fig. 2D), suggesting that conversion of PUFA to MUFA was triggered. Meanwhile, Gpam (p<0.05, 48.20-fold) and Dgat (Diglyceride acyltransferase, 2.654-fold) mRNA levels were significantly higher (p<0.05) in carcass grade 1++/1+ than in carcass of grade 1 (Fig. 2E and 2F), suggesting that TG synthesis was promoted.
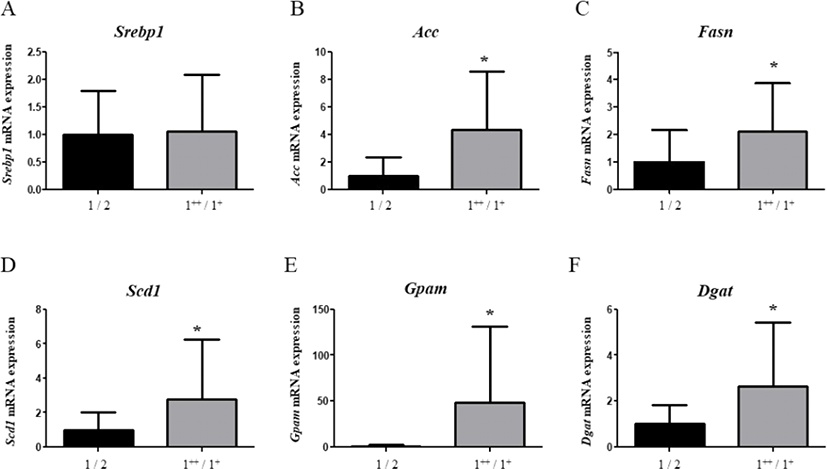
As glycolysis is known to be promoted in high grade carcasses, we next evaluated the mRNA levels of representative glycolytic genes. As a result, mRNA levels of Hk1 (Hexokinase1, 2.66-fold) and Pfk (Phosphofructokinase, 2.26-fold) were significantly higher (p<0.05) in carcass grade 1++/1+ than in carcass of grade 1 (Fig. 3A and 3B). However, there were no significant differences in the level of Pk (Pyruvate kinase) mRNA between groups (Fig. 3C). These results suggest that glycolysis is promoted above carcass grade 1.
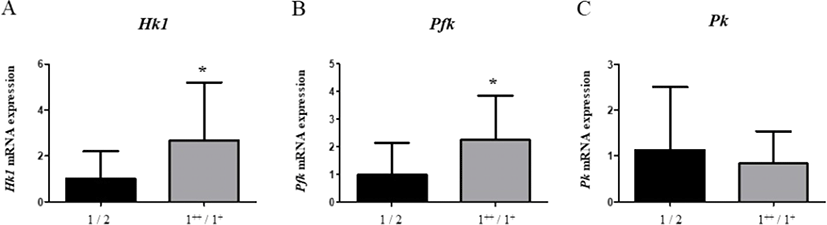
To evaluate the effect of Pgrmc1 on carcass grade, we evaluated mRNA levels of Pgrmc1. Data were provided for 52 Hanwoo livers. As a result, the level of Pgrmc1 mRNA in grade grade 1++/1+ carcasses was significantly lower (11%, p<0.05) than the levels in grade 1 carcass (Fig. 4A). Moreover, levels of Pgrmc1 mRNA in carcass grade 1/2 were higher (p = 0.0169) than those in grade 1++/1+ carcasses (Fig. 4B).
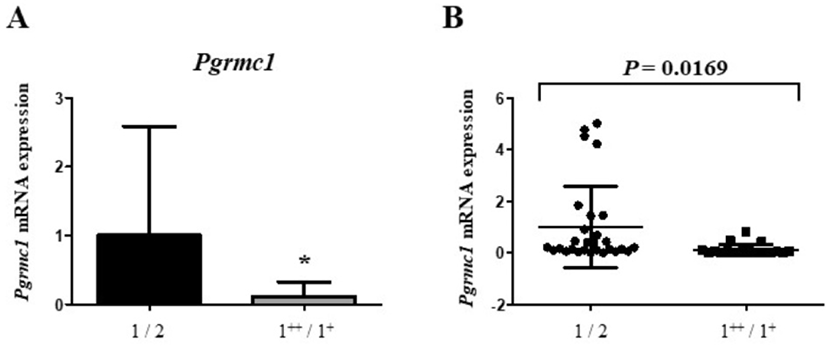
As the correlation between PGRMC1 and lipogenesis in vitro has already been studied [12], we focused on the relationship between PGRMC1 and glycolysis in the present study. Among glycolysis genes, Hk1 and Pfk levels were higher in higher grade carcasses, so their mRNA levels were analyzed in the Hep3B cell line. While PGRMC1 level was suppressed (55%), levels of HK1 (2.89-fold) and PFK (2.9-fold) were significantly higher in the PGRMC1 siRNA group compared to the Control siRNA group (p<0.05, Fig. 5A–C), suggesting that glycolysis was induced after PGRMC1 knockdown.
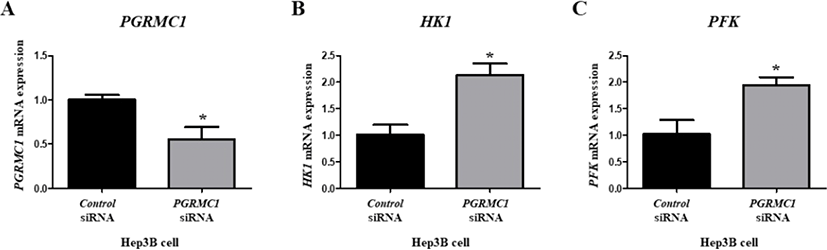
Discussion
The weight of Hanwoo carcasses is lower on average than that of the carcasses of US breeds of the same age at time of slaughter, suggesting less fattiness in Hanwoo carcasses than in US breeds. Intramuscular fat is affected by fattiness and is called marbling, which is a major factor in determining carcass grade [13]. When cattle take up nutrients from carbohydrate- or fat-rich diets, their livers synthesize fat using fatty acids and DNL. As a major contributor in DNL, SREBP-1 promotes visceral fat mass and MUFA levels [14]. Interestingly, Hanwoo possess high levels of MUFA due to lipogenesis in the liver.
SREBP-1 is classified into SREBP-1 and SREBP-2. While SREBP-2 plays a role in cholesterol synthesis, SREBP-1 triggers fatty acid synthesis. SREBP-1 normally exists in precursor form in the ER along with INSIG-1 and SCAP and is activated by a high carbohydrate diet. SREBP-1 then goes into the nucleus and becomes mature, called nuclear SREBP-1. Nuclear SREBP-1 can act as a transcriptional regulator for several genes, especially those related to fatty acid synthesis and TG synthesis [15]. As loss of PGRMC1 protein induces structural instability of the SREBP-1 precursor, SREBP-1 precursors are travel through the Golgi complex to the nucleus. In consequence, loss of PGRMC1 triggers lipogenesis by inducing SREBP-1 activation [12]. Therefore, the decreased Pgrmc1 mRNA levels in carcasses grade 1++/1+ suggest a decrease in PGRMC1 protein levels and an increase in SREBP-1 mature protein levels. Though Srebp1 mRNA levels did not show significant differences, as PGRMC1 mainly influences SREBP1 protein rather than mRNA, our results are consistent with those of previous studies. Unfortunately, we could not evaluate the levels of SREBP-1 protein because of lack of host reactivity. However, mRNA levels of Srebp-1 transcriptional genes suggest that decreased Pgrmc1 levels caused promotion of lipogenesis and TG accumulation in liver.
In DNL, a series of steps causing lipogenesis, starts with acetyl-CoA which is then carboxylated by ACC. ACC is therefore a rate-limiting step in fatty acid synthesis. The product from acetyl-CoA is malonyl-CoA. Malonyl CoA is further saturated by several enzymes and finally, lipogenesis is terminated by FASN [16]. Therefore, it can be suggested that lipogenesis is induced in carcass grades 1++/1+ because expression levels of two crucial enzymes for lipogenesis, Acc and Fasn, are increased. These two enzymes are also transcriptionally regulated by SREBP-1. Moreover, the present study is emphasized by previous studies suggesting that FASN can be used as superior genetic modification of Hanwoo [17]. After lipogenesis, SFA is desaturated into MUFA by SCD1 and is used for TG synthesis. While SCD1 is also transcriptionally regulated by SREBP-1, SCD1 overexpression is linked to metabolic disorders such as obesity and diabetes [18]. In cattle, Scd1 can also be used as genetic modification of Hanwoo. It is important because MUFA produced by SCD1 is a key factor in the flavor and tenderness of beef [19].
After fatty acid desaturation, MUFA combines with glycerol backbones in de novo TG synthesis. In TG synthesis, there are four steps including the enzymes GPAM, 1-acylglycerol-3-phosphate-O-acyltransferase (AGPAT), Phosphatidate phosphatase (PAP), and DGAT. GPAM, and DGAT are associated with the first and last steps of TG synthesis as they join fatty acyl CoA to glycerol backbones. In detail, GPAM is rate-limiting step for TG synthesis and DGAT determines flux into TG [20, 21]. More importantly, DGAT and GPAM have been suggested as candidate genes for intramuscular fat deposition in cattle [22, 23].
In DNL, acetyl CoA is synthesized from pyruvate, which is synthesized through the glycolysis pathway. In glycolysis, there are 10 steps including three irreversible steps. Three enzymes are involved in the irreversible steps: HK1, PFK, and PK. Among them, PFK is the most crucial enzyme as it controls the rate-limiting step of glycolysis [24]. By producing more pyruvate through the glycolytic pathway, it is highly likely that it is used for fatty acid synthesis in high carcass grades in the present study. Furthermore, we observed induction of glycolysis genes after PGRMC1 knockdown in vitro in Hep3B cells. Through this, we confirmed that PGRMC1 suppression leads to abundance of pyruvate sources that can be used for lipogenesis.
In conclusion, we found significant difference in Pgrmc1 mRNA level according to carcass grade. We suggest Pgrmc1 as a valuable novel marker for producing high carcass grade cattle, which is closely related to DNL, TG synthesis, and glycolysis in the liver. The results of the present study can be used for genetic improvements in carcass grades of cattle. Since Pgrmc1 can be detected in blood peripheral nucleated cells, it also has value for rapid blood diagnosis [25]. In further studies, it will be valuable to monitor the relation between level of Pgrmc1 in blood and carcass grade in muscle. The selected cow expressing low Pgrmc1 will be used for genetic improvement.