Introduction
Colorectal cancer (CRC) is a major health problem in developed Western countries. According to Cancer Statistics in Korea, the mortality rate per 100,000 population due to cancer is 150.9 and take the first place in 2014 [1]. Among all cancers, the mortality rate due to colon cancer and diagnostic rate of adenomatous polyp, a biomarker of colon cancer incidence, has increased [1, 2]. Incidence of CRC is linked to conditions such as diet, physical activity, obesity and smoking, etc [4]. There are still some controversy surrounding red meat consumption and CRC. Western style diet high in red and processed meat is risk factor for colon cancer [5]. Several hypotheses could explain how red meat and processed meat can increase CRC risk. Pro-cancer factors in red meat might be excess fat, excess protein, excess iron, or heat-induced mutagens [6]. Many evidences support the hypothesis that excess iron is associated with increased risk of CRC [5, 7, 8].
Iron itself is a vital trace element in life. However, iron not only enable to activate vital enzymes through gain ing and losing electrons, but also participate in potentially deleterious free radical-generating reactions. The Fenton reaction, in which ferrous iron donates an electron in a reaction with hydrogen peroxide to produce the hydroxyl radical, produces reactive oxygen species (ROS) [9]. ROS may interact with and modify cellular protein, lipid, and DNA, thereby resulting in altered target cell function. The resultant oxidative stress leads to the extension and propagation of crypt abscesses, either through direct membrane disruption by lipid peroxidation or through generation of secondary toxic oxidants such as chloramines [10]. Oxidative DNA damage may participate in ROS-induced carcinogenesis [11]. Hydroxyl radicals are believed to promote tumor development by causing chromosomal damage. There are two distinct routes by which colonic tissue is exposed to iron; one is passing through gastrointestinal tract (exogenous) and another is body iron storage and serum iron level effecting on colon tissue (endogenous) [12]. Thus, excess iron intake and inappropriate iron administration may interfere with normal body defenses and promote cancer [13]. Oxidative stress from excess ROS formation can be reduced by antioxidant scavenging or detoxifying excess ROS. Therefore, therapeutic interventions with antioxidants can be useful for chemoprevention of cancers [14].
In colon, single epithelial cell suffers histogenetic change and gradual proliferation and then form morphologically distinct foci as a first stage of colonic aberrant crypt foci (ACF) formation [15]. ACF represent the earliest step in colorectal carcinogenesis [16]. ACF undergo multi-step event to form polyps as one of endpoint biomarkers of CRC [17]. Among animal CRC model, chemically induced CRC model by using azoxymethane (AOM) shows large, thick and dark colonic crypt growth [18]. The combination of a single hit of AOM with 1 week exposure to the inflammatory agent dextran sodium sulphate (DSS) in rodents has proven to dramatically shorten the latency time for induction of CRC and to rapidly recapitulate the aberrant crypt foci–adenoma– carcinoma sequence [19].
Meanwhile, ascorbic acid (AA) reduces oxidative stress as an antioxidant. However, it maintains iron and other transient metal in a reduced state, leading to produce ROS formation in vitro and enhance bioavailability of non-heme iron, leading to greater body iron storage. Although AA with iron does not increase oxidative stress in vivo [20], effect of AA in CRC needs to be investigated.
There are no studies about iron-overload effect with AA in CRC animal model. This study was carried out to investigate the effect of iron-overload with/without AA on the formation of pre-neoplastic lesions induced by AOM/ DSS in ICR male mice
Materials and Methods
AOM was obtained from Sigma-Aldrich Inc. (St. Louis, MO). DSS, whose molecular weight is 36,000~50,000, was obtained from MP Biomedical Inc.. Fe2O3 (>95%) was obtained from Sigma-Aldrich Inc.(Santa Ana, CA). Ascorbic acid was obtained from Junsei Inc. (Tokyo, Japan).
Male ICR mice (4 weeks old) were obtained from Koatech Inc. (Pyeongtaek, Korea) and housed in an isolating polycarbonate cage (5 mice/cage). The temperature and relative humidity were maintained at 20 ± 2 °C and 50 ± 20%. Light and dark cycles were at 12 h each, and the intensity of illumination was maintained at 150-300 lux. The AIN-76A purified diet from Central Animal Laboratory Inc. (Seoul, Korea) was given to the mice. During the experimental periods, diets and litter were all used after sterilization, and the animal experiment was conducted in compliance with “Guide for care and use of Laboratory animals”of Chungbuk National University (IACUC approval Number: CBNUA-812-15-01). During the experimental period, body weight and feed consumption were recorded once a week.
After acclimation for 1 week, five-week old mice were divided into 4 groups (10 mice/group) including 1) control, 2) AA, 3) Fe, and 4) Fe + AA groups. AIN-76A purified diet (34.25 Fe mg/kg diet) and distilled water were supplied to the mice. Carboxymethylcellulose (CMC) as a dissolution agent was orally administrated to the control group. Fe2O3 (450 mg/kg b.w.) and/or AA (450 mg/ kg b.w.) mixed with 1% CMC were also orally administrated to the designated groups. The mice were intraperitoneally treated with AOM (10 mg/kg) three times at 0, 1st and 2nd weeks of the experimental period to induce the formation of pre-neoplastic lesion in colon. After the last injection of AOM, distilled water with 2% DSS was provided for 7 days, and the total experimental period is 6 weeks (Fig. 1).
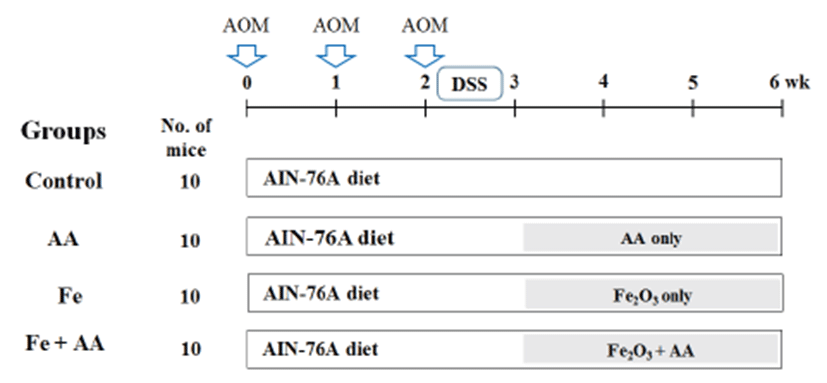
After finishing administration for 6 weeks, all mice were sacrificed. After anesthesia and laparotomy, blood was collected by a syringe from the abdominal aorta. The liver and entire large intestine were harvested and then frozen in liquid nitrogen (-196 °C). The large intestine was washed with saline and fixed flat in 10% neutral buffered formalin.
The formalin-fixed colonic tissues were stained in 0.5% methylene blue solution for 30 sec, and the total number of ACF was counted under a microscope (40-100x), and the number of polyps was counted by an unassisted eye. The total number of ACF and the number of polyps were recorded as number/colon.
For the determination of total iron, samples of liver were analyzed by inductively coupled plasma emission spectrophotometer (ICP-OES) (OPTIMA 7300DV, Perkin Elmer, Waltham, MA). Frozen samples were digested and ashed at 200°C for 4 h using concentrated nitric acid and hydrogen peroxide. For the ICP-OES analysis of iron, the digested sample was diluted with the equal amount of de-ionized water before analysis.
TBARS assay was conducted for determination of malondialdehyde (MDA) which is a by-product of lipid peroxidation in the serum and liver. At first, collected sample 100 μL, 8.1% sodium dodecyl sulfate (SDS) solution 100 μL, and 20% acetic acid solution 200 μL were mixed together, and 0.75% 2-thibarbituric acid (TBA) solution 100 μL was added lastly, and they were vortexed and spined down. Then, the incubation at 95°C was conducted for 30 min, and the centrifuge process was conducted at 4°C, 13,000 rpm for 15 min. At last, they were put in the 96-well plate, and the optical density of the supernatant liquid was measured at 532 nm. The protein quantitative analysis was conducted with using DC Protein kit (Bio-rad Laboratories, Inc., Hercules, CA) which was applied by the Lowry method.
Results
The mice body weights of all experimental groups were increased with the passage of time (Fig. 2). Fe group showed a decrease in body weight during 4-5th week of experimental period but it had nearly the same body weight at 6th week of the experimental periods compared with control and other groups. There was no significant difference among experimental groups.
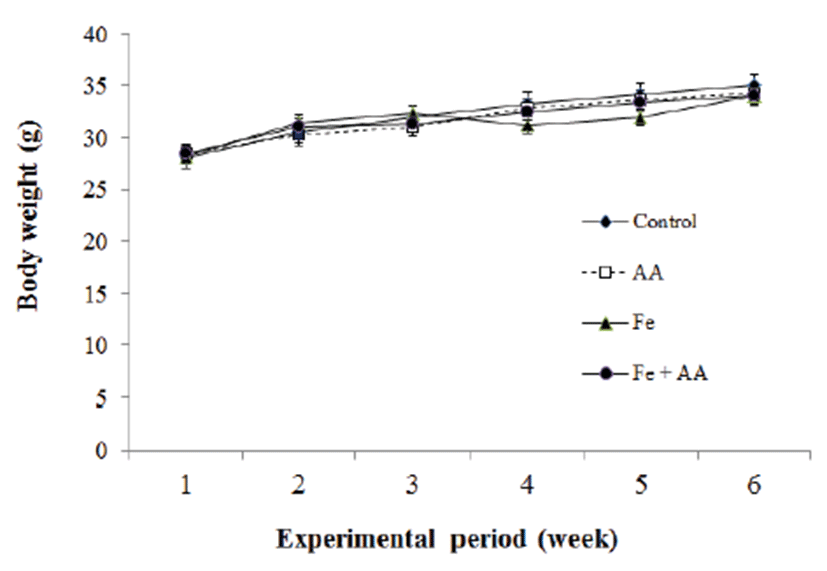
Iron store in the liver was measured by an ICP-OES. Fe group and Fe + AA group had significantly higher concentration of iron in the liver of mice than the control group or AA group (p<0.05) (Fig. 3). Moreover, AA treatment significantly increased the iron concentration of liver between the iron-treated groups (p<0.05) (Fig. 3).
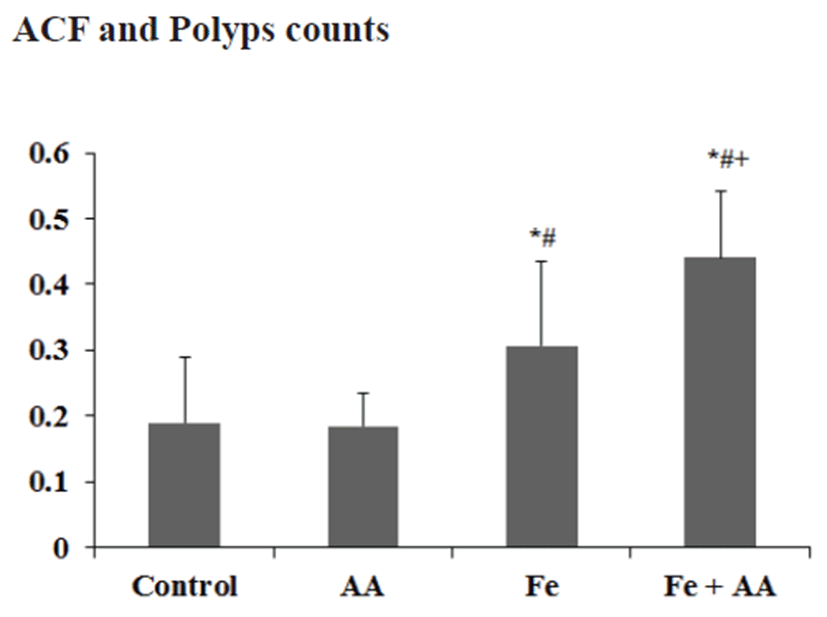
The numbers of ACF/colon in the control, AA, Fe, and Fe +AA groups were almost similar (Fig. 4). There was no significant difference between them (Fig. 4). The numbers of polyps/colon in Fe-treated groups were a little bit higher than those of the Fe non-treated groups, and AA treatment did not affect the polyps formation in the colon of mice (Fig. 5). There were no significant differences among experimental groups (Fig. 5).
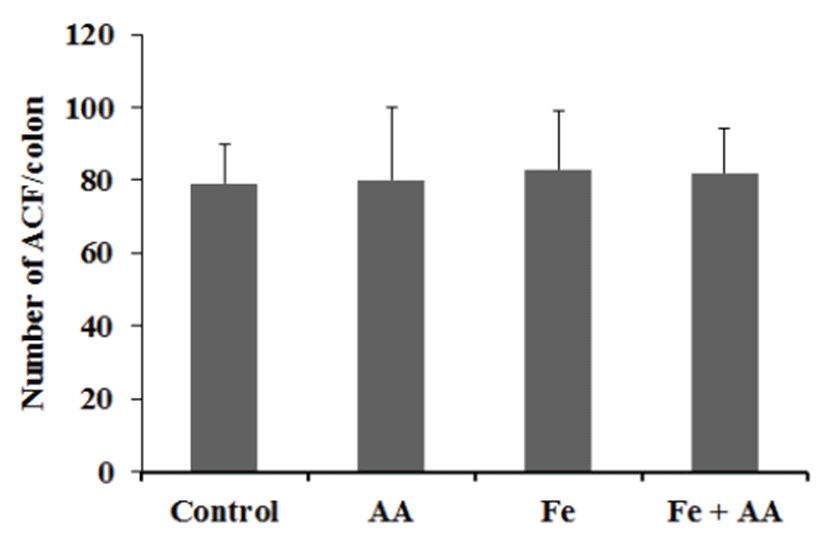
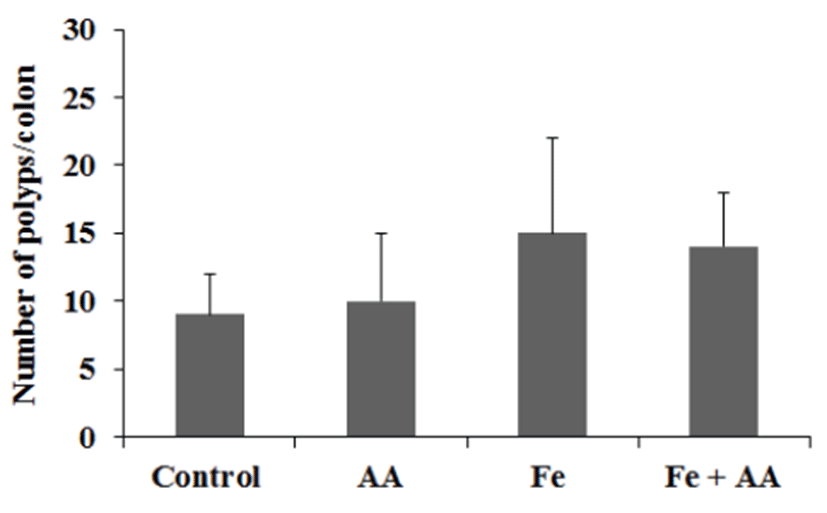
In serum, the TBARS values of Fe group and Fe + AA group were slightly higher than those of control group or AA group, but no significance was founded between them (Fig. 6). In liver, the TBARS values of Fe group and MFe + AA group were significantly higher than those of the control group and AA group (p<0.05) (Fig. 7). There was no significant difference between Fe-treated groups.
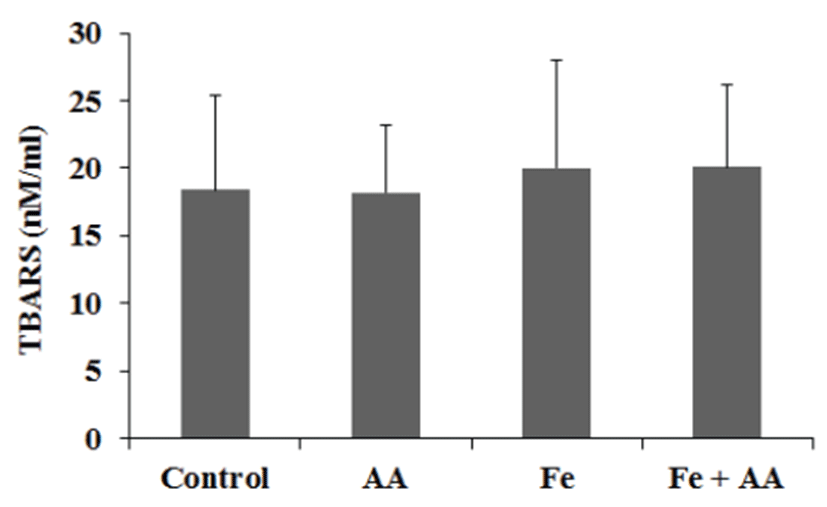
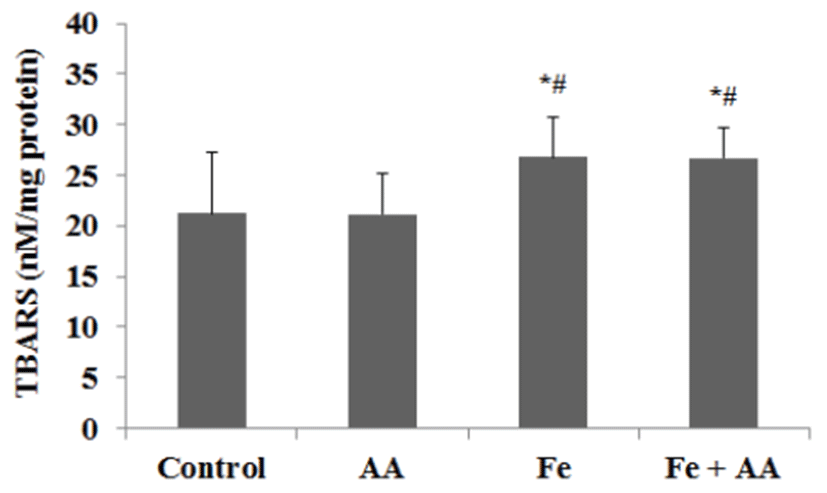
Discussion
The objective of the present study was to determine an effect of overloaded iron with AA on colon carcinogenesis as evaluated by colonic ACF induced by AOM in male ICR mice. The mice were fed on AIN-76A purified diets and distilled water, and Fe (450 ppm, Fe2O3) and/or AA (450 ppm) mixed with 1% CMC were administrated to mice in the designated groups daily.
In the present study, iron overload with ascorbic acid did not affect the formation of pre-neoplastic lesion in the experimental colon carcinogenesis. These findings may not be inconsistent with other studies, in which iron increased oxidative stress and pre-neoplastic lesion in CRC [14, 20]. AA could reduce lipid peroxidation in liver under certain conditions [20]. Our results could not explain the effect of iron and AA on colonic pre-neoplastic lesion in other animal model [7, 8].
Liver iron contents increased in all iron-treated groups, especially in Fe + AA group. The result corresponds well with those found in the earlier studies [12, 13]. Lipid peroxidation in liver comparable to iron content in liver confirmed that liver iron contents caused oxidative damage to liver [21]. In addition, increased iron contents in liver did not correspond to incidence of colonic pre-neoplastic lesion. This result resembles that the effect on colonic pre-neoplastic lesion from body iron storage was less specific than from intestinal luminal iron [22], but is not in accord with reports that liver iron contents affect several cancers [23, 24]. On the other hand, there is another report that inorganic iron (ferric citrate) does not affect incidence or promotion of CRC but increases the size of lesion [25]. But there is little agreement with this study. To argue that opinion would carry us too far away from the purpose of this study.
In the present study, AA did not affect the decrease in the formation of pre-neoplastic lesion unlike other study [26]. But iron with AA showed increased liver iron contents and similar level of lipid peroxidation despite of higher liver iron contents than iron alone. This result may be explained by previous studies [20].
Interestingly, increases of lipid peroxidation in all irontreated groups were higher in liver than in serum. It may be due to iron-catalyzed lipid peroxidation by high iron contents in liver. Meanwhile, lipid peroxidation in serum could not be increased easily because of tightly bounded ferric iron state in serum.
Furthermore, as measured by the number of polyps, promotion rates of colonic pre-neoplastic lesion in all irontreated groups were higher than those in other groups, although there were no significant differences among experimental groups.
Although this study is not enough to explain the fuller aspect of iron with AA on the formation of colonic preneoplastic lesion, our results would give some basic information for the study for colon carcinogenesis. Further study will be required to ascertain effect of iron and AA in several colorectal carcinogenesis models.