Introduction
Tissue injury results in inflammatory nociception, and various inflammatory mediators such as prostaglandins (PGs), leukotriens, histamine, bradykinin, and plateletactivating factor are involved in initiating and sustaining nociception and the inflammatory cascade [1, 2]. The classical symptoms of acute inflammation are fever, redness, swelling, and pain caused by increased blood flow. In inflammatory responses, arachidonic acid is transformed into PGs via the cyclooxygenase (COX) pathway [3]. Symptoms of the inflammation process can be alleviated by non-steroidal anti-inflammatory drugs (NSAIDs), which mainly inhibit COX and reduce synthesis of PGs, and by corticosteroids, which prevent the formation of PGs by inhibition of phospholipase A2 (responsible for arachidonic acid release) [4]. However, both steroidal and NSAID medications can have undesirable side effects including gastric erosions [5]. Therefore, there is currently great interest in natural compounds that have been used for centuries to reduce pain and inflammation with few side effects.
Vitis species from the family Vitaceae have traditionally been used world-wide as medicinal herbs. Grape and its bioactive constituents have various pharmacological effects, including antioxidant, antibacterial, anticancer, anti-inflammatory and antidiabetic activities, as well as hepatoprotective, cardioprotective and neuroprotective effects [6]. Vitis amurensis is one of the most common wild grapes in Korea, Japan, and China. Bioactive compounds such as Vam3, resveratrol, and procyanidins from its root, stem, and seeds were found to have anti-inflammatory properties in in vitro experimental models [7-9].
Aralia species (Araliaceae) have long been recognized as therapeutic herbs with antinociceptive, antidiabetic, antioxidant, and anti-inflammatory activities. The roots of Aralia cordata have been shown to protect inflamma-tory arthritis in rabbits [10]. In a previous study, we found anti-nociceptive and anti-inflammatory effects of the leaves and stems of Aralia cordata [11]. Potential antiinflammatory candidates such as diterpenes, triterpenes, saponins, sterols, and polyacetylenes were isolated from the roots, leaves and stems of Aralia cordata [12, 13].
Glycyrrhizae radix, the root of Glycyrrhiza uralensis, has beneficial effects in brains such as memory enhancing effects [14, 15] and protection of ischemic brain damage in animal models [16]. These pharmacological activities are because of its anti-inflammatory ingredients, which include glycyrrhetinic acid, glycyrrhizic acid, and liquiritigenin [17-19].
In previous studies, the leaves and stems of Vitis amurensis, the aerial part of Aralia cordata, and Glycyrrhizae radix showed neuroprotection against amyloid β proteininduced and ischemic brain damage because of their anti-oxidant and anti-apoptotic effects [15, 20-23]. Furthermore, preparations that combined these three plants (SSB), have been demonstrated to have neuroprotective effects against amyloid β protein-induced neurotoxicity in mice [11] and ischemia-induced rat brain damage [24] via a synergistic effect. Since inflammation is a part of the complex series of pathophysiological events that contribute to neurodegenerative diseases such as Alzheimer's disease and stroke, it was hypothesized that neuroprotection of the SSB may be associated with its anti-inflammatory activity. The present study was conducted to investigate the properties of anti-nociception as well as anti-inflammation of SSB by employing chemical and thermal pain stimuli and formalin-induced paw inflammation in mice.
Materials and Methods
The leaves and stems of Vitis amurensis and the aerial parts of Aralia cordata were collected at Keryong Mountain in Daejeon, Korea, from May to July and Glycyrrhizae radix (Korean) was purchased from Daegu Oriental Pharm Co., Daegu, Korea. These plants were identified by Dr. Kyung-Sik Song, Kyungpook National University. Two-hundred grams of each of the three plants was mixed, extracted with ethanol (3 L × 24 h × 3) at room temperature, filtered, and concentrated under reduced pressure using a rotary evaporator to yield an ethanol extract (SSB, 64 g), which was stored at room temperature until required. Ibuprofen was purchased from Sigma Chemical Co. (St. Louis, MO, USA), and morphine was acquired from Myungmoon Pharm. Co. (Gyunggi-Do, Korea).
Male ICR mice (5 weeks old) were supplied by Samtako, Inc. (Gyunggi-Do, Korea) and housed in an environmentally controlled room at 22 ± 2°C under a relative humidity of 55 ± 5% and a 12 h light/dark cycle, while provided with food and water ad libitum. The procedures involving experimental animals complied with the Animal Care Guidelines of the National Institutes of Health and the Animal Ethics Committee of Chungbuk National University.
Acetic acid (0.8%) was intraperitoneally injected into mice 20 min after the administration of vehicle (normal saline, p.o.), SSB (25, 50, and 100 mg/kg, p.o.), ibuprofen (100 mg/kg, p.o.), or morphine (5 mg/kg, s.c.). Each mouse was then placed in an individual clear plastic observation chamber, and the total number of writhes made by each mouse was counted between 10 and 40 min after acetic acid administration. The counts of writhing response in the control group were regarded as 100%. For scoring purposes, a writhe was indicated by stretching of the abdomen with simultaneous stretching of at least one hind limb [25].
The test was conducted using a Hot Plate Analgesia Meter (IITC Life Science, Woodland Hills, CA, USA). The hot plate temperature was kept constant at 55 ± 1°C. The mice were gently placed on the hot plate and jumping with all four paws was considered as nociception. Only mice showing a pretreatment reaction time ≤ 5 s were selected. The mice were only kept on the hot surface for 20 s (cut-off time) to avoid any thermal injury [26]. The reaction time of jumping was measured before and repeatedly at different times (15, 30, 45, 60 and 90 min) after administration of vehicle (normal saline, p.o.), SSB (25, 50, and 100 mg/kg, p.o.), ibuprofen (100 mg/kg, p.o.), or morphine (5 mg/kg, s.c.).
Mice were placed in an open Plexiglas observation chamber for 30 min to acclimate to their surroundings, after which they were gently restrained while 20 μL of 5% formalin solution in saline was administered subcutaneously to the dorsal surface of the right hind paw using a 30 gauge needle [27]. Following formalin injection, mice were immediately returned to the observation chamber for a 30 min observation period. The time in seconds spent licking the injected paw as the nociceptive response for both the first neurogenic phase (0–5 min) and second inflammatory phase (20–30 min) was record ed with a chronometer [28]. Mice were treated with SSB (25, 50, and 100 mg/kg, p.o.), vehicle (normal saline, p.o.), ibuprofen (200 mg/kg, p.o.), or morphine (5 mg/ kg, s.c.) 30 min before formalin.
The same formalin-injected mice were utilized for measurement of paw edema volume. The vertical paw thickness of the injected paw and the contralateral noninjected paw was measured at the metatarsal level as the index of paw edema using a fine caliper (Vernier caliper 530 series, Mitutoyo, Japan) 18 h after formalin injection. Paw edema was calculated by subtracting the paw thickness of the contralateral non-injected side from that of the formalin-injected side and expressed as mm thickness [29].
Data were expressed as the means ± S.E.M. and statistical significance was assessed by the one-way analysis of variance (ANOVA) followed by Tukey's test. All statistical analyses were performed using Prism GraphPad v.5.0 (La Jolla, CA, USA). P values of <0.05 were considered to be significant.
Results
SSB (25, 50, and 100 mg/kg) significantly reduced acetic acid-induced writhing response to 53.6 ± 7.9, 45.2 ± 7.1 and 27.0 ± 5.3%, respectively. Ibuprofen (100 mg/kg) reduced the acetic acid-induced writhes to 40.4 ± 7.0%, and morphine (5 mg/kg) completely inhibited them (Fig. 1).
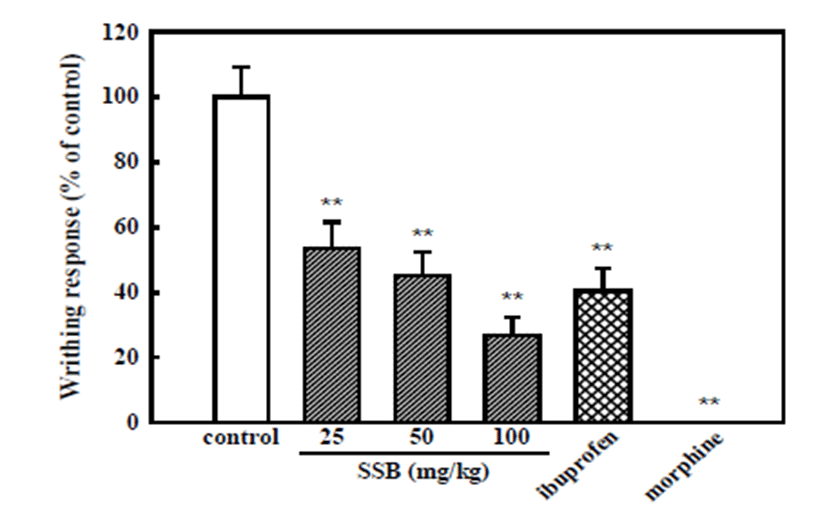
As shown in Fig. 2, SSB and ibuprofen did not increase the latency period of pain induced by nociceptive stimuli by the hot plate. These results indicate that SSB as well as ibuprofen did not exert anti-nociceptive effects on thermal stimuli-induced algesia. In contrast, the opioid drug morphine (5 mg/kg) significantly prolonged the latency of escape from the hot plate at 15, 30, 45, 60, and 90 min after its administration, indicating anti-nociceptive activity.
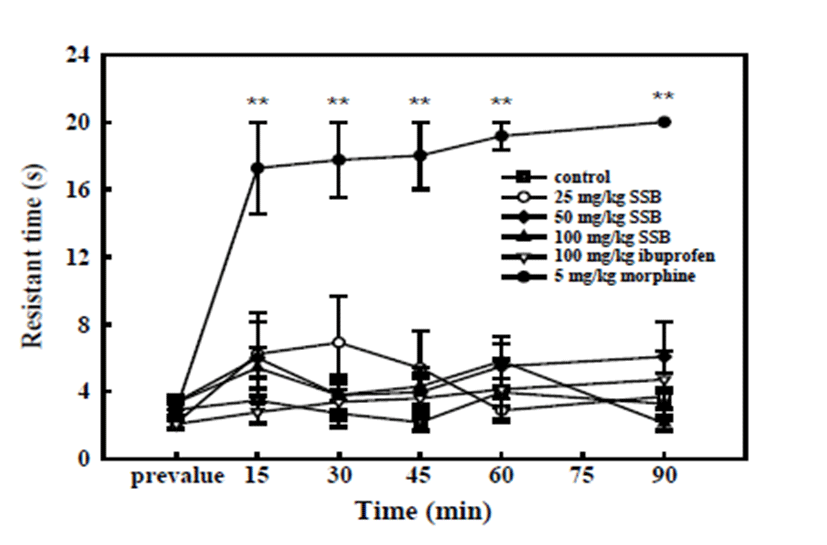
SSB (50 and 100 mg/kg) and ibuprofen (200 mg/kg) significantly decreased formalin-induced licking time relative to the vehicle-control (99.0 ± 8.9 s) in the second inflammatory phase (20–30 min), with values of 69.5 ± 4.6, 38.9 ± 7.6 and 52.3 ± 10.9 s, respectively, being observed. However, neither SSB nor ibuprofen inhibited the first phase of neurogenic nociception. Meanwhile, morphine (5 mg/kg) significantly inhibited both the first neurogenic and the second inflammatory nociception induced by formalin injection (Fig. 3).
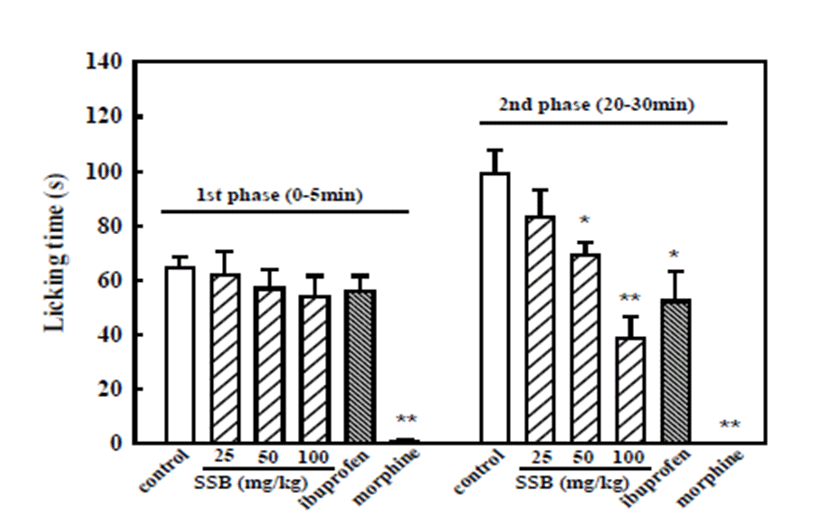
Subcutaneous injection of formalin to the dorsal sur-face of the paw elicits significant inflammation (e.g., paw edema) [29, 30]. To investigate whether the apparent attenuation of formalin-induced nociceptive behavior during the second phase reflected an inhibitory effect of SSB on the peripheral inflammation produced by formalin injection, the change in thickness of the hind paw edema was compared between vehicle-treated and SSB-administered mice. As shown in Fig. 4, SSB (25, 50, and 100 mg/kg) and ibuprofen (200 mg/kg) significantly inhibited formalin-induced paw edema, showing 0.48 ± 0.06, 0.43 ± 0.06, 0.37 ± 0.07, and 0.36 ± 0.06 mm paw thickness, respectively, when compared with the control group (0.72 ± 0.03 mm, thickness).
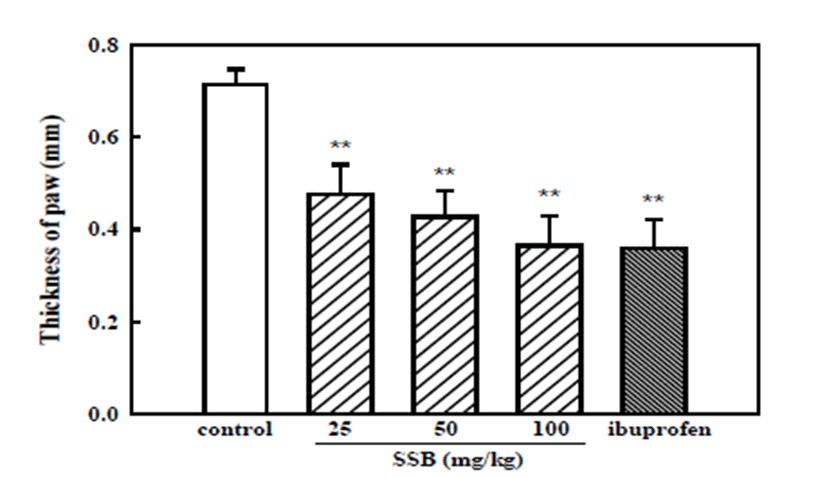
Discussion
The acetic acid-induced writhing test is a typical model for measurement of inflammatory pain that is commonly used to screen new agents with peripheral anti-nociceptive and anti-inflammatory properties [31]. Intraperitoneally injected acetic acid causes tissue damage and triggers the liberation of a variety of mediators such as bradykinin and PGs, as well as some cytokines such as TNF-α, IL-1β, and IL-8 [32, 33]. PGs are generated during metabolism of arachidonic acid by the COX pathway and activate chemosensitive nociceptors that contribute to the development of inflammatory pain [3]. Thus, NSAIDs such as ibuprofen or indomethacin, COX inhibitors, are commonly used as analgesics and anti-inflammatory agents [2]. SSB as well as ibuprofen used as a reference NSAID significantly inhibited the acetic acid-induced writhing response, suggesting a mechanism involved in the anti-nociceptive effect by the inhibition of mediator release of the inflammatory process in mice.
The hot plate test using thermal stimuli is employed to investigate the anti-nociceptive activity mediated by central mechanisms observed in opioid-like analgesics, while peripheral compounds are inactive in this kind of painful stimulus [34]. The involvement of endogenous substances such as PGs may be minimized in this model [35]. The hot plate test was performed to determine whether the activity of the SSB on the acetic acid-induced writhing test was associated with central anti-nociceptive activity or not. While morphine increased the escape latency from the hot plate, both SSB and ibuprofen failed to increase the escape latency. This result supported that anti-nociceptive activity of SSB in the acetic acid-induced writhing test may be related to a peripheral mechanism.
Unlike traditional reflex tests of nociception such as tailflick and hot-plate, pain produced by the hind paw injection of formalin results from persistent tissue damage and thus more closely resembles clinical pain conditions [29]. Subcutaneous injection of formalin into the back of the hind paw produces a distinct biphasic response of the first phase, which begins at the time of injection and lasts for about 10 min, and a second phase, which starts at 10 min postinjection and lasts for about 50 min. The first phase is considered to be a direct result of stimulation of nociceptors in the paw that reflects centrally mediated pain. The second phase is pain due to inflammation with a release of serotonin, histamine, bradykinin, and PGs. As a result, different analgesics may act differently in the first and second phases of this test; therefore, this test can be used to clarify the possible mechanisms of an anti- nociceptive effect of a test compound [36, 37]. Centrally acting drugs such as opioids inhibit both phases equally, but peripherally acting drugs such as NSAIDs and corticosteroids only inhibit the second phase [37, 38]. In the present study, the first and the second phase responses were observed 0–5 min and 20–30 min after formalin injection, respectively. Pretreatment orally with SSB (50 and 100 mg/kg) showed a significant inhibitory effect on the licking activity in the second phase, but not in the first phase. Similarly, ibuprofen produced a marked inhibition of the inflammatory nociception of the second phase only. However, morphine inhibited both phases of the formalin test. These results suggested that the anti-nociceptive effect of the SSB was specific to the inflammatory pain, possibly through inhibition of PGs synthesis, but not to the neurogenic pain.
Paw injection of formalin in rodents causes local tissue injury of the paw, which results in inflammatory pain and edema [37]. Its main characteristics are the exudation of fluid and plasma proteins and the emigration of leukocytes, predominantly neutrophils, caused by vasoactive biogenic amines such as bradykinin, histamine, serotonin and PGs [30]. SSB as well as ibuprofen showed significant inhibition of formalin-induced paw edema, further supporting that SSB could inhibit peripheral inflammatory pain in the present study.
In previous reports, we demonstrated an anti-nociceptive and anti-inflammatory effect and inhibitory effect on COX-1 and COX-2 of the leaves and stems of Aralia cordata and isolated diterpenes, triterpenes, and a saponin as active constituents of the pharmacological activity [11, 13]. Studies demonstrated a variety of anti-inflammatory components from Vitis amurensis and Glycyrrhizae radix, suggesting anti-inflammatory effect of these plants [8, 9, 17, 19]. However, no previous studies have investigated the anti-nociceptive and anti-inflammatory effects of the leaves and stems of Vitis amurensis and Glycyrrhizae radix. Mice treated with 50 mg/kg SSB showed 45.2 ± 7.1% of the writhes observed in control mice, whereas mice treated with 200 mg/kg Vitis amurensis and Glycyrrhizae radix showed 49.9 ± 19.4% and 59.1 ± 14.9%, respectively, (data not shown). In a previous study, the leaves and stems of Aralia cordata at 200 mg/kg resulted in 54.7 ± 5.0% of the writhes observed in a control [11]. Therefore, the results of the current study indicate that the three medicinal plants acted synergistically to produce anti-nociceptive and anti-inflammatory activity. In conclusion, these results suggest that SSB exerted anti-nociceptive and anti-inflammatory effects. The antinociceptive effects of SSB may be mediated through its anti-inflammatory action through inhibition of inflammatory mediators such as PGs. Overall, this study supports a potential use of SSB as a therapeutic agent for the alleviation of inflammation and inflammatory pain.