Introduction
Wound healing is a fundamental response to tissue injury. Healing involves epithelialization, granulation, and tissue remodeling caused by inflammatory responses [1]. Several innate substances are known to promote wound healing [2]. Among them, β-glucan is a fiber-type complex sugar (polysaccharide) found on cell walls of baker’s yeast, oat and barley fibers, and many medicinal mushrooms. β-glucan promotes immune system activity [3, 4] and lowers blood cholesterol levels [5, 6]. Similar to other polysaccharides [7-9], β-glucan has been reported to accelerate wound healing [10-12]. Previously, we showed that β-glucan can stimulate proliferation and migration of human dermal fibroblast cells in a in vitro fibroblast populated collagen lattice (FPCL) system mediated by TGF-β [13] as well as promote full-thickness wound healing in an infection murine model [14]. However, the in vivo effect of β-glucan on wound healing under diabetic conditions has not yet been evaluated. For this reason, the stimulatory effect of β-glucan on dermal wound healing should be assessed in a diabetic animal model, which displays delayed wound healing.
Therefore, the objective of the present study was to examine the effects of β-glucan originating from Aureobasidium on full-thickness skin wound healing in diabetic C57BL/KsJ-db/db mouse models.
Materials and Methods
One hundred male genetically diabetic C57BL/KsJ-db/db mice (7-wk old upon receipt, Jackson Laboratory, USA) were used after 7 days of acclimatization. Five animals were allocated per polycarbonate cage in a temperature (20~25°C) and humidity (45~50%) controlled room. Light:dark cycle was 12 hr:12 hr and feed (Samyang, Korea) and water were supplied free to access. All mice were wounded, and about half of the animals were selected 1 day after wounding according to body weight, blood glucose level, and wound size. All laboratory animals were treated according to the national regulations of the usage and welfare of laboratory animals, and the procedure was approved by the Kyungpook National University Experimental Animal Ethical Committee.
For detecting blood glucose levels, blood samples were collected from wounds at the orbital plexus and deposited into NaF glucose vacuum tubes (Becton Dickinson, USA), after which plasma was separated. Blood glucose levels were detected using an automated blood analyzer (Toshiba 200 FR, Japan) (Table 2).
Full-thickness dermatotomies (round; 10 mm) were prepared on the shaved dorsal wall of the diabetic mice. One day after wounding, about half of the animals showing similar wound areas were selected.
β-glucan was purified from Aureobasidium pullulans SM-2001, which is a UV-induced mutant of A. pullulans [15]. MadecassolTM (Dongkook Pharm. Co., Korea) was used in this study. β-glucan (2.5% solution) was diluted in distilled water and topically applied at dosages of 10, 50, and 100 mg/kg and at a volume of 10 ml/kg starting from 1 day after wounding, twice a day for 20 days. In addition, MadecassolTM was also topically applied at 100 mg/kg, twice a day for 20 days. The administered dose and schedule of these drugs are shown in Table 1. β-glucan were sterilized at 121°C for 10 min before use.
Group | Dose | |
---|---|---|
Control | Vehicle only | |
|
||
Test groups | β-glucan | 10 mg/kg |
β-glucan | 50 mg/kg | |
β-glucan | 100 mg/kg | |
|
||
MadecassolTM | 100 mg/kg |
Changes in body weight were calculated at 0, 2, 4, 8, 12, 16, and 20 days after test article application. In addition, body weight gains were calculated as follows (Equation 1).
Wound areas were measured on the initial applied day (just immediate before test article application) as well as 2, 4, 8, 12, 16, and 20 days after test article application after wounding as mm2 using an automated image analyzer (analySIS Image Processing; SIS, Germany) attached to a stereoscope. The 50% contraction time (CT50) was calculated using probit methods, and WS % changes (% changes in wound area between wounding and sacrifice) were calculated as follows (Equation 2).
After measuring last wound sizes, wounded area of skin containing dermis was sampled. Sampled skin was fixed in 10% neutral-buffered formalin. After paraffin embedding, 3~4 μm sections were prepared. Representative sections were stained with Hematoxylin and Eosin (H&E) for light microscopic examination, after which the histological profiles of individual skin were observed. For histomorphometric evaluation, the numbers of inflammatory cells such as polymorphonuclear cells (PMNs), macrophages, lymphocytes, and fibroblasts in granulation tissues (N/mm2) were counted at 21 days after wounding using automated image analysis (analySIS Image Processing; SIS, Germany). The number of micro-vessels in granulation tissues (N/mm2) was counted at 21 days after wounding using automated image analysis. The degree of re-epithelialization was estimated as a percent (%) of the re-epithelialized filled length in each wound tissue using automated image analysis. (Table 2)
All data were calculated as mean ± S.D. (n=10). Statistical analyses were conducted using Mann-Whitney U-Wilcoxon Rank Sum W test (MW test), and CT50 was calculated based on measured wound sizes using Probit methods with SPSS for Windows (Release 6.1.3., SPSS Inc., USA). Inhibition rates compared to that of vehicle control were calculated to determine the effects of test materials on differences between control and test groups (Equation 3).
Results
There were no significant changes in body weight among all tested groups compared to the control, except for slight decreases in all test article applied groups (Table 3).
Body weight during experimental periods showed that it underwent changes of – 6.15, – 7.08, – 9.31, and – 6.52% in the madecassol and 10, 50, and 100 mg/kg of β-glucan groups compared with control group, respectively.
Wound size (WS) significantly decreased in a dose-dependent manner in a β-glucan-treated groups compared to the control over a treatment period of 16 or 20 days (p<0.01 or p<0.05). Wound size was significantly reduced at β-glucan concentrations of 50 and 100 mg/kg starting from day 8 when MadecassolTM had no effect. Wound size also significantly decreased in the madecassol group starting from day 12 (p<0.01 or p<0.05). The reducing effect of β-glucan on wound size was stronger than that of MadecassolTM. In addition, changes (%) in wound size significantly increased among all tested groups compared to the control (p<0.01 or p<0.05). There were also significant decreases in CT50 (p<0.01) (Table 4).
There were dose-dependent increases of angiogenesis, re-epithelialization, and reconstruction of the dermis in all β-glucan groups compared to the control. Except for angiogenesis, skin wounds showed similar reconstruction between the madecassol-treated group and β-glucan-treated groups (Fig. 1). There were dose-dependent and significant decreases in the numbers of inflammatory cells such as PMNs, macrophages, and lymphocytes in the 50 and 100 mg/kg of β-glucan groups compared to the control (p<0.01). Further, there were significant increases in the numbers of micro-vessels and fibroblasts as well as the rate of re-epithelialization (p<0.01). In the 10 mg/kg of β-glucan group, significant (p<0.01 or p<0.05) reduction of inflammatory cells and elevation of fibroblasts were observed compared to the control. In the madecassol-applied group, similar changes in inflammatory cells and fibroblasts were observed with a significant (p<0.01) increase in re-epithelialization, although no meaningful changes were observed in angiogenesis (Table 5).
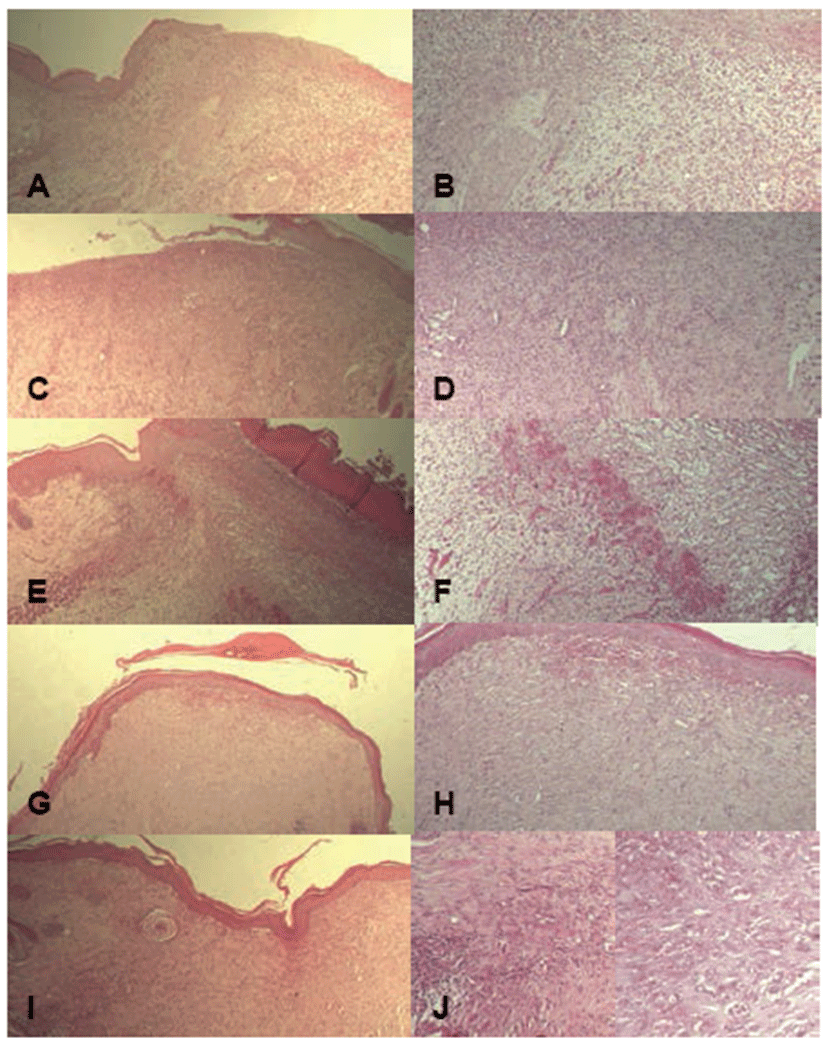
Discussion
In the present study, the direct effects of β-glucan on wound healing in diabetic mice were examined for the first time. As a result of treatment with β-glucan, significant (p<0.01 or p<0.05) and dose-dependent decreases in wound size were observed compared to the vehicle control showing increased WS%. In addition, CT50 was dramatically and dose-dependently reduced, and inflammatory cells in granulation tissues of wound areas were also significantly (p<0.01 or p<0.05) and dose-dependently decreased compared to the vehicle control showing increased numbers of micro-vessels and fibroblasts as well as re-epithelialization. In the madecassol-applied group, similar changes in inflammatory cells and fibroblasts with re-epithelialization were observed, although MadecassolTM did not affect angiogenesis. These data suggest that β-glucan has properties that may be beneficial for wound healing, especially for intractable bedsores and other chronic ulcers frequently encountered in diabetic patients.
The C57BL/KsJ-db/db mouse is a mutant diabetic mouse model showing a delayed healing response in the dermis relative to normal mice [16]. These db/db mice also show obesity and hyperglycemia at 5-wk-old [17, 18]. In the present study, db/db mice showed hyperglycemia and obesity before wounding. No meaningful changes in body weight or gain were detected in the present study, except for a non-significant and slight decrease in body weight gain in all tested groups compared to the control. This result can be attributed to the stress of relatively long term (20 days) application of test articles, which means that topical application of β-glucan did not show any serious toxicity up to 100 mg/kg in diabetic mice.
In the present study, β-glucan potentially increased contraction of wounds in diabetic mice similar to normal mice. Increased wound contractions are considered as a basic characteristic of wound management agents. Reduction of CT50 and elevation of WS% detected in the β-glucan-applied groups can be considered as a direct evidence that β-glucan promoted wound contraction in diabetic mice.
Wound healing involves overlapping steps of inflammation, cell migration and proliferation, neovascularisation, and extracellular matrix production and remodeling, and collagen is a major component of the extracellular matrix [19]. Inflammation followed by tissue repair is a complex physiological process aimed at restoration of normal function after infection or wounding [20, 21]. Advances in growth factor discovery, biochemistry of the extracellular matrix, and immunology have enhanced our knowledge of inflammation and wound healing, including angiogenesis. While inflammation stimulates the production of angiogenic growth factors, angiogenesis is an independent event from inflammation [22, 23]. Angiogenesis occurs during later stages of tissue repair and is essential to restoration of tissue damaged by injury and inflammation [23-25]. Though many growth factors possess angiogenic properties and are commercially available in recombinant forms, their widespread clinical use is hindered by their prohibitive costs [26]. In addition, their half-lives in the bloodstream are likely to be short and unpredictable due to the presence of a variety of binding proteins. Therefore, identification and characterization of alternative compounds actively promoting wound healing are important to the development of new therapeutic strategies. Dose-dependent decreases in inflammatory cells such as PMNs, macrophages, and lymphocytes were detected in β-glucan-applied groups showing increased numbers of micro-vessels and fibro blasts as well as re-epithelialization in the present study. This result can be considered as direct evidence that β-glucan promoted wound reconstruction from granulation tissues in diabetic mice, similar to normal mice. Although the β-glucan used in this study did not influence collagen production of fibroblasts in vitro, it is possible that collagen production increased in vivo as a secondary effect of increased proliferation of fibroblast-mediated TGF-β1. In this study, dramatic increases in fibrosis and fibroblast numbers were observed.
The mechanism of action of β-glucan in the promotion of wound healing is complex. The anti-inflammatory activities of β-glucan in this study might be beneficial for dermal wound healing, similar to the effect of polysaccharide extract from Phellinus gilvus [27]. In addition, β-glucans are potent macrophage stimulators that enhance macrophage cytotoxicity and phagocytic capacity [28, 29]. Leibovich and Ross [30] reported that wound healing is delayed when wound macrophages are depleted. Therefore, it is possible that dermal wound healing may be promoted by the macrophage activity of β-glucan. Further, promotion of wound healing by β-glucan also stimulated fibroblasts and its specific cytokine TGF-β1 since proliferation of fibroblasts is promoted by β-glucan-mediated TGF-β1.
These data suggest that β-glucan has properties that may be beneficial for wound healing, as it promoted contraction of wounds and reconstruction of the dermis and epidermis. These data show that β-glucan has enough wound healing effects to be developed as a wound management agent or as an adjuvant for the treatment of skin wounds, especially intractable bedsores and other chronic ulcers frequently encountered in diabetic patients. However, additional studies regarding its mechanism of action will reveal its usefulness and limitations.