Introduction
Colorectal cancer is one of the leading causes of cancer-related deaths in Korea and many other developed countries [1]. Malignant tumors such as colon cancer are considered to be complications of chronic inflammatory diseases [2]. A new field in chemoprevention research has discovered molecular links between inflammation and cancer. Control of inflammation is widely accepted as an important strategy for cancer chemoprevention. Cyclooxygenase-2 (COX-2) and inducible nitric oxide synthase (iNOS) are critical enzymes that are up-regulated in most chronic inflammatory diseases and cancers, including ulcerative colitis and colon cancer [3]. Numerous studies have revealed that COX-2 and iNOS play important roles in the development of cancers and are especially associated with functions in tumor angiogenesis, cell growth and metastasis, enhanced cellular adhesion, and inhibition of apoptosis [4, 5, 6]. It has been reported that COX-2 is overexpressed in cancers affecting the lungs, prostate, and large intestines as well as in cancer cell lines [7, 8, 9]. It was also widely reported that suppression of inflammatory activity by selective COX-2 inhibitors can reduce incidence of colorectal cancers by inhibiting the action of prostaglandins [10, 11]. COX-2 and iNOS are induced by various inflammatory stimuli [12]. Some animal studies on selective COX-2 or iNOS inhibitors have demonstrated suppressed colorectal carcinogenesis [13, 14, 15]. Therefore, it is widely accepted that the chemopreventive effects of COX-2 or iNOS inhibitors on cancer development are due to anti-inflammation through inhibition of COX-2 and iNOS activities [16, 17, 18].
Aberrant crypt foci (ACF) are considered to be pre-neoplastic lesions and putative biomarkers of colorectal cancer [19]. Several studies have reported that COX-2 and iNOS are up-regulated in ACF and colorectal carcinogenesis in animals and/or humans [6, 19, 20]. Carcinogen-induced ACF and colitis-related colon cancer rodent models are frequently used to investigate colon cancer development and screen potential chemopreventive agents [17, 18].
Alpha-viniferin (AVF), a resveratrol trimer, is an oligomeric stilbene purified from Caragana chamlague Lamarck and Carex humilis Leyss [21, 22]. Resveratrol (3,4’,5-trihydroxystilbene) is a phytoalexin polyphenolic compound present in grapes and wines that has been shown to inhibit diverse cellular events associated with proliferation of many cancer cell lines as well as suppress development of carcinogen-induced mammary and colorectal neoplasms in animal models [23, 24]. The chemopreventive effect of resveratrol is known to be partly due to enhanced tumor cell apoptosis [24, 25]. AVF shows an anti-inflammatory effect in cotton oil-induced paw edema model in vivo [10]. The anti-inflammatory mechanism of AVF may be related to its inhibitory effects on COX-2 and iNOS in paw edema or macrophage cells [10, 21]. In addition to its anti-inflammatory effects, AVF has been reported to inhibit acetylcholinesterase, protein kinase C, and tyrosinase activities as well as keratinocyte proliferation [21].
Whereas the anti-inflammatory and cancer chemopreventive effects of resveratrol have been well documented in numerous in vitro and in vivo studies, there has been no report on the effects of AVF regarding suppression of cancer development. Thus, we tried to investigate the cancer chemopreventative effects of AVF in colorectal carcinogenesis in vitro and in vivo.
Materials and Methods
All animal experimental procedures were approved by the Animal Use and Care Committee of College of Veterinary Medicine, Chungbuk National University. Male ICR mice were purchased from Damul Inc. (Daejeon, Korea) at 5 weeks of age and housed in plastic cages at 24 ± 2°C under 55% relative humidity and a 12-hr light-dark cycle. Water and basal diet were given ad libitum. AVF was chemically synthesized at the College of Pharmacy of Chungbuk National University. AOM was purchased from Sigma Chemical Co. (St. Louis, MO). DSS with a molecular weight of 40,000 was purchased from ICM Biochemicals Inc. (Aurora, OH).
The human colon carcinoma cell line Caco-2 was cultured in Dulbecco’s modified Eagle’s medium (DMEM) (Gibco) supplemented with 10% FBS (Gibco), 100 μg/ mL of streptomycin, 100 U/mL of penicillin, and 0.1 mM non-essential amino acids and maintained at 37°C with 5% CO2.
Caco-2 cells were pre-treated with AVF compound for 24 hrs. After autopsy, colon tumor tissues from mice were washed with saline. Cytoplasmic extracts of cells were resolved by SDS-acrylamide gel electrophoresis and transferred onto polyvinylidene difluoride membranes. Membranes were incubated with anti-COX2 (1:500), anti- iNOS (1:1000), and anti-caspase-3 antibody (1:1000) at 4°C for 16 hrs. After incubation with horseradish peroxidase-conjugated anti-rabbit IgG antibody (1:2500) at room temperature for 3 hrs, blots were treated with ECL reagents (Amersham-Pharmacia) and then exposed to X-ray films.
Caco-2 cells were washed with PBS and then fixed with 4% paraformaldehyde at 4°C for 1 hr. Cells were permeabilized with 0.1% Triton X-100 at room temperature for 10 min and incubated with blocking solution (containing 0.1% BSA) for 15 min. Cells were incubated with rabbit anti-COX2, anti-iNOS, and anti-caspase-3 antibodies in PBS (1:500) containing 5% BSA at 4°C overnight, followed by incubation at room temperature with secondary antibodies in PBS (1:200) containing 5% BSA for 1 hr in the dark. Fluorescence was observed by a fluorescence microscope (Zeiss).
Cells were fixed in 4% paraformaldehyde in PBS for 1 hr at room temperature and washed with PBS containing 0.1% Triton X-100. Cells were washed three times with PBS and incubated in a TUNEL reaction mixture containing terminal deoxynucleotidyl transferase and a fluoresce-labeled nucleotide mixture (F-dUTP) (In situ cell death detection kit; Roche Molecular Biochemicals, Germany). Briefly, each group of cells was incubated with 20 μL of the TUNEL reaction mixture containing 2 μL of enzyme solution and 18 μL of labeling solution for 1 hr at 37°C in the dark. After incubation, cells were washed with PBS and analyzed by a confocal scanning laser fluorescence microscope (Model FV300, Olympus).
Forty-five male mice were randomly divided into three groups: AOM-induced mice without AVF (AOM group), AOM-induced mice treated with AVF low dose (AOM + LD), and AOM-induced mice treated with AVF high dose (AOM + HD). AOM was treated once a week for 3 weeks intraperitoneally at a dose of 10 mg/kg. AVF dissolved in water was orally administered at doses of 0.05 (LD) or 0.1 mg/mouse (HD) from one day before initial AOM treatment until the end of this experiment. All mice were sacrificed at 5 weeks after first AOM treatment. After autopsy, entire large intestines were excised, filled with 10% neutral-buffered formalin, and then opened longitudinally. Each colon was then fixed flat between sheets of filter paper on 10% neutral-buffered formalin. Fixed colons were dipped in 0.2% methylene blue in water for 45 seconds and then placed on a glass slide with the mucosal surface up. The number of aberrant crypt foci was counted using a light microscope with a 4-fold magnification objective lens. TABLE 1, 2
Treatment | Mice with ACFs | ACFs/colona | AC/focusa* |
---|---|---|---|
AOM | 15/15 | 44 ± 12.2 | 1.4 ± 0.1 |
AOM + LD | 15/15 | 32 ± 8.5 | 1.4 ± 0.1 |
AOM + HD | 15/15 | 28 ± 9.4* | 1.4 ± 0.1 |
Treatment | No. of mice with tumor (%) | Tumors/mousea | Total no. of tumor mass | AD /ADCAb |
---|---|---|---|---|
Control | 18/22 (82) | 3.6 ± 0.72 | 81 | 10/8 |
LD | 17/22 (77) | 2.9 ± 0.57 | 65 | 11/6 |
HD | 14/22 (63)** | 1.8 ± 0.49* | 40** | 9/5 |
Sixty-six male mice were randomly divided into three groups (control, low dose (LD) group, and high dose (HD) group). AVF dissolved in water was orally administered at doses of 0, 0.05 (LD) or 0.1 mg/mouse (HD) for 28 weeks. The next day, all mice received a single i.p. injection of AOM at a dose of 10 mg/kg. One week later, mice were exposed to 2% DSS in drinking water for 7 days. All mice were sacrificed at the 28th week. After autopsy, the large bowels were flushed with saline and opened longitudinally to measure the number and sizes of tumor masses. The tumor tissues were fixed in 10% buffered formalin for 24 hrs and routinely processed and embedded in paraffin for histopathology.
Immunohistochemistry against COX-2 and iNOS in pre-neoplastic tissues as well as COX-2, iNOS, and caspase-3 in tumor tissues was performed. Paraffin-embedded sections (5 μm) were deparaffinized, rehydrated, treated with 0.3% hydrogen peroxide for 15 min to quench endogenous peroxidase activity, and then rinsed briefly in PBS. The sections were covered with 2% normal serum for 20 min to block non-specific binding. Then, sections were incubated overnight with anti-COX2 and anti-cleaved caspase-3. After rinsing with PBS, sections were incubated according to the manufacturer (Vectastain Elite ABC kit; Vector Laboratories). Reaction products were developed by immersing sections in 3,3’-diaminobezidine 4HCl solution (Sigma) containing 0.1% hydrogen peroxide.
Results
To examine the modulating effects of AVF on COX-2, caspase-3, and iNOS expression in Caco-2 colonic cancer cells, Western blot analysis was carried out. The proteins were detectable in resting Caco-2 cells (Fig. 1A). Treatment with AVF (100 μm) significantly decreased the expression levels of COX-2 (P<0.05) and iNOS protein. In addition, caspase-3 expression was significantly up-regulated by AVF compared to the control group (Fig. 1B).
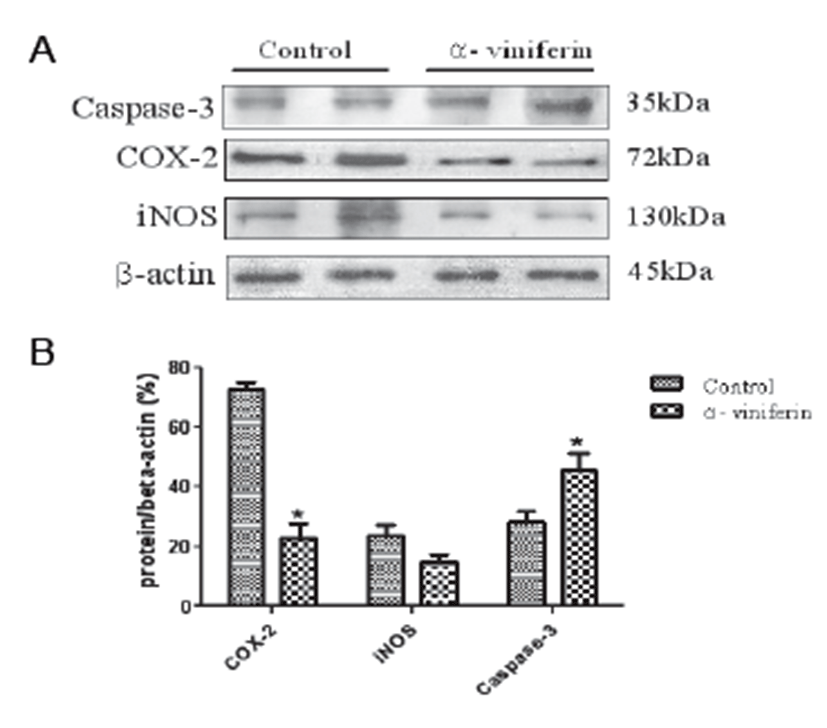
Immunofluorescence analysis demonstrated that COX-2, caspase-3, and iNOS expression levels were modulated by AVF in Caco-2 cells (Fig. 2). Expression levels of COX-2 (Fig. 2A) and iNOS (Fig. 2B) were reduced by AVF compared to untreated Caco-2 cells. As demonstrated in Fig. 2C, AVF treatment (100 μm) for 24 hrs induced typical changes in caspase-3 expression pattern. Caspase-3 expression increased in Caco-2 cells treated with AVF compared to control cells (Figs. 2C, panels b and e). However, its expression was not detected in nuclei of Caco-2 cells.
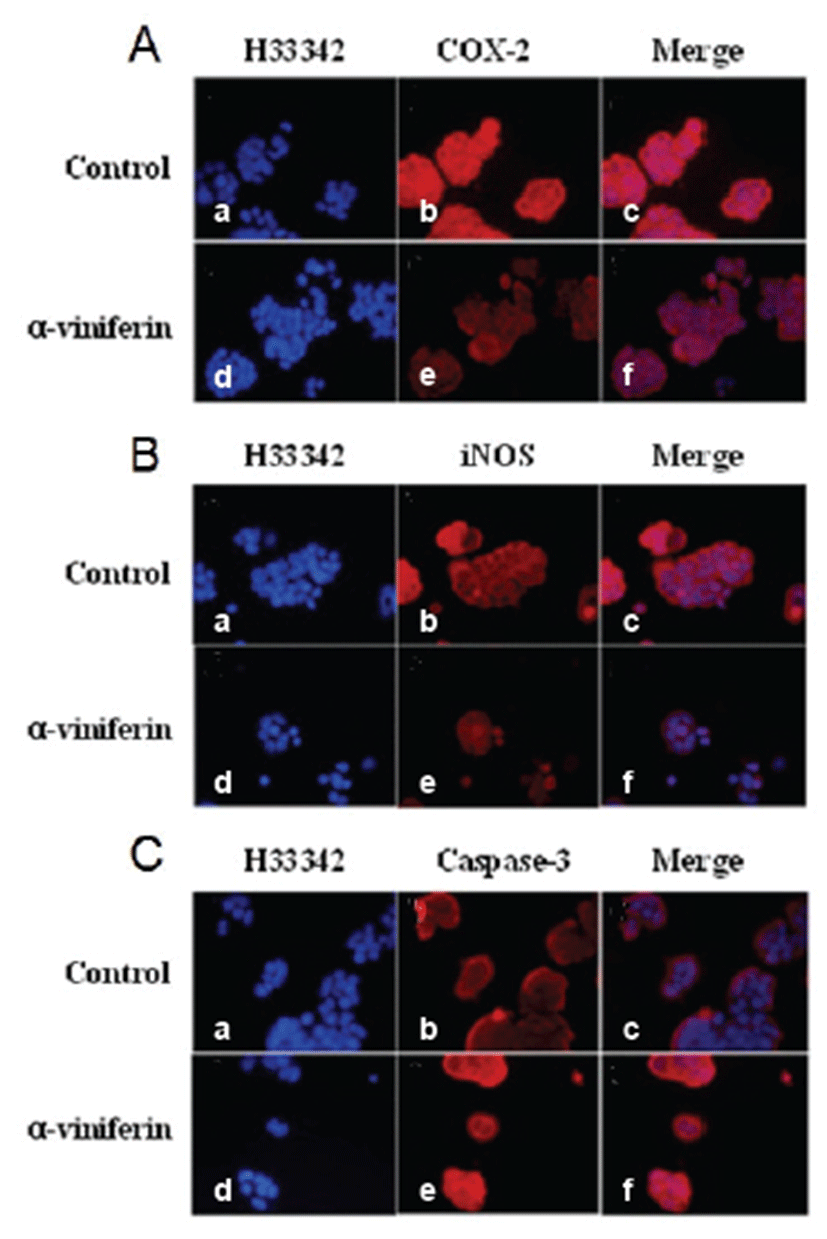
We examined the pro-apoptotic activity of AVF in Caco- 2 cells (Fig. 3). In untreated cells, apoptosis was weakly observed by TUNEL assay (Fig. 3B, green), whereas apoptosis increased upon AVF treatment (Fig. 3E, green).
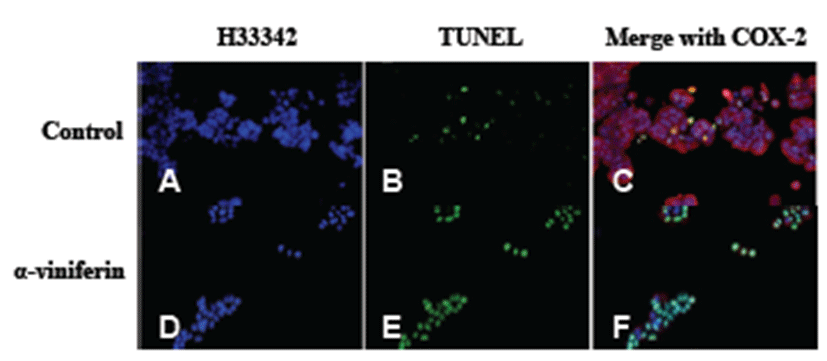
Table 1 summarizes the data on ACF development. ACF were observed in colon tissues of all mice treated with AOM. Administration of AVF to AOM-treated mice reduced the number of ACF in a dose-dependent manner to 73% and 64% of the control value in the AOM + LD group and AOM + HD group, respectively. In the AOM + HD group, the reduction was significant (P<0.05). The mean number of aberrant crypts (ACs) per focus in AOM-treated mice was unchanged. To examine the suppressive effect of AVF on COX-2 and iNOS, immunohistochemistry was performed in pre-neoplastic lesions. In AOM-treated colon tissue, COX-2 was localized mainly in stromal cells of the mucosa (Fig. 4A), whereas iNOS expression was detected in the cytoplasm of epithelial cells (Fig. 4B). Expression of proteins was suppressed by AVF treatment (Figs.4C-4F).
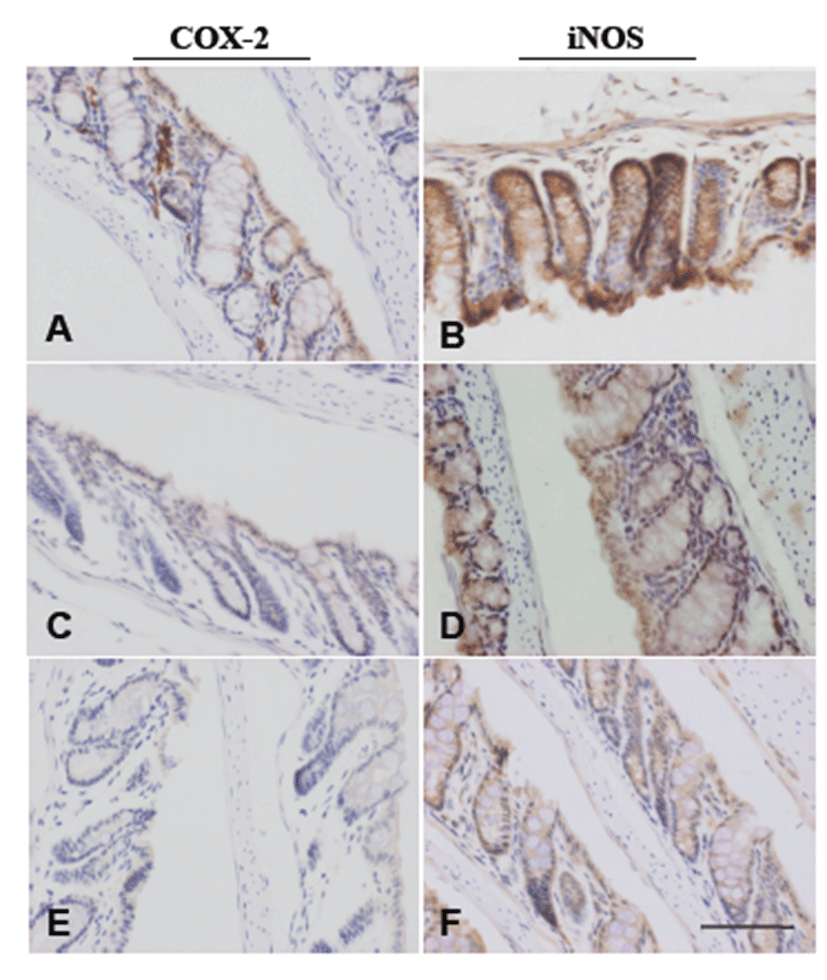
Tumor incidence and histopathological classification are summarized in Table 2. Colorectal tumors were located mainly in the distal and middle colons in all groups. AVF treatment significantly decreased the number of tumors. In the control group, incidence was 82%, whereas 77% and 63% incidences were detected in the LD and HD groups, respectively (P<0.005). The total number of tumors and number of tumors per mouse significantly decreased in the HD group (P<0.005). However, according to histopathological classification of the tumors, the adenoma/adenocarcinoma ratio was not affected by AVF treatment. Immunohistochemical localizations of COX-2, iNOS, and caspase-3 showed similar patterns as in Caco-2 cells (Fig. 5). Likewise, in pre-neoplastic tissues, iNOS was localized to epithelial cells (Figs. 5C and 5c) while COX-2 was in stromal cells (Fig.5A) from tumor tissues of control mice. Expression of iNOS and COX-2 was reduced by AVF administration (Figs. 5D and 5F). Caspase-3 was strongly expressed in epithelial cells and stromal cells (Fig. 5B). AVF administration enhanced the expression of caspase-3 diffusely (Fig. 5E).
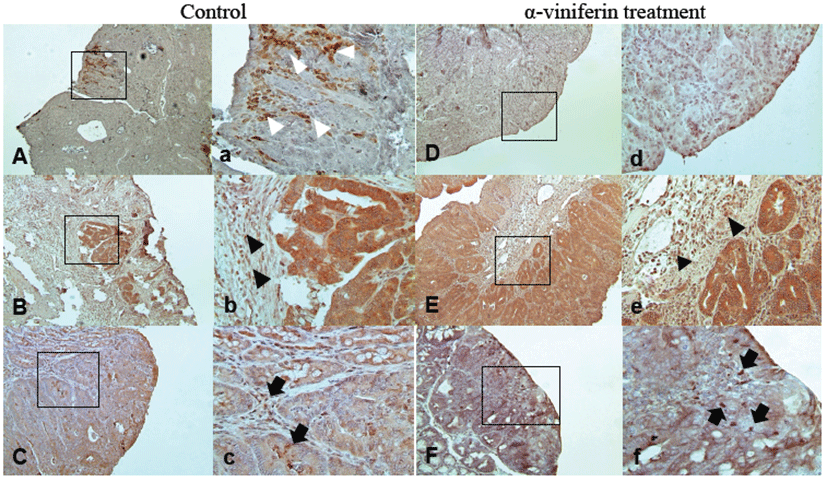
Discussion
The aim of this study was to examine the chemopreventive potential of AVF in colonic carcinogenesis. In this study, we investigated the relationship between inhibition of COX-2 and iNOS and apoptosis induction by AVF in Caco-2 human colorectal cancer cells. In addition, we tried to examine the chemopreventive effects of AVF on ACF formation and colitis-related colorectal tumorigenesis in a mouse model. We found that AVF treatment caused reduction of colorectal tumor development by suppression of inflammatory enzymes COX-2 and iNOS and pro-apoptotic activity.
Several studies have shown that resveratrol has many biological and pharmacological effects, including cancer chemopreventive and anti-inflammatory activities [24, 25, 26]. Resveratrol exerts inhibitory effects on diverse cellular events associated with tumor initiation, progression, and promotion of cancer cells [27]. In line with these reports, AVF as a trimer of resveratrol likewise shows anti-inflammatory activities [21]. In this study, AVF down-regulated COX-2 and iNOS levels in Caco-2 cells as well as mouse colorectal tumorigenesis. Moreover, we observed the pro-apoptotic effects of AVF in vitro and in vivo. Therefore, our results support the hypothesis that AVF may have similar effects as resveratrol in terms of chemopreventive potential via anti-inflammatory action.
The earliest steps of colorectal carcinogenesis have been shown to be associated with ACF formation in the form of pre-neoplastic lesions along with over-expression of COX-2 and iNOS in a rodent model [10, 15, 28]. Therefore, the present study demonstrated that AVF significantly suppressed AOM-induced ACF formation in mice. In other reports, when COX-2 was inactivated, the numbers and sizes of colorectal polyps significantly decreased in a adenomatous polyposis model [29]. Using immunohistochemistry or immunofluorescence, we found that AVF inhibited expression of COX-2 and iNOS in Caco-2 cells and chemically-induced pre-neoplastic and neoplastic tissues. Furthermore, AVF activated apoptosis of colorectal cancer cells by activating caspase-3 enzyme, which plays critical roles in execution of cellular apoptosis. Expression patterns of COX-2, iNOS, and caspase-3 as determined by immunohistochemistry were similar to other investigations.
In conclusion, AVF showed suppressive effects on growth of colorectal tumors induced by azoxymethane and DSS in mice. The effects were due to its inhibitory effects on COX-2 and iNOS and enhancement of apoptosis via caspase-3 activation. Therefore, AVF may be a candidate chemopreventive agent for treatment of colorectal carcinogenesis, although further investigations are necessary to understand the exact mechanism of AVF.