Introduction
Vertebrate ectoderm is differentiated into neural plate, neural plate border and non-neuronal ectoderm after gastrulation. The neural plate border, which consists of neural crest cells and cranial placode cells, is located between the non-neural ectoderm and the neural plate. The neural crest cells and the neural placodes delaminate from the epithelial structure from which they originate, and they have the ability to differentiate into a large arByungray of cell types including sensory neurons, neuroendocrine cells, glia and supporting cells [1]. Neural crest and placodes share a number of important features pointing to a possible common evolutionary origin [2]. It has been suggested that neural crest and placodes may have evolved from a common ancestral population present in vertebrate ancestors [3].
The development of neural crest and placode involves morphological changes of cells, and both neural crest and placodes develop from populations of cells near the border of the neural plate [4]. The neural crest is a multipotent population of cells that is characteristic of vertebrate embryos posterior to the diencephalon. Several types of placodes can be distinguished at the neural border: adenohypophysial placode, olfactory placode, lens placode, profundal placode, trigeminal placode, a series of epibranchial placodes contributing to the distal ganglia of the facial, glossopharyngeal, and vagal nerves, hypobranchial placodes, a series of lateral line placodes giving rise to the lateral line ganglia and lateral line organs, and the otic placode [5-7]. Among the cranial placodes, the otic placode is particularly remarkable because the inner ear and the vestibulocochlear ganglion develop from it. The induction and development of the otic placode has long been a popular subject of experimental embryology studies because it is readily accessible and undergoes such a complex morphogenesis [8].
The Sox proteins constitute a large array of transcription factors [9]. Among the Sox family members, Sox9 regulate the chondrogenesis in mouse embryos [10, 11]. Mutation of Sox9 results in Campomelic Dysplasia and severe skeletal malformations [12-14]. Sox9 is an essential component for the regulation of neural crest formation and a key regulator of inner ear specification in Xenopus [15]. Morpholino mediated knockdown of Sox9 generated embryos exhibiting a specific loss of earlyneural crest markers [16]. Wnt signaling regulates the induction of neural crest progenitors depending on the functions of Sox9 as Wnt mediated neural crest induction is inhibited in the context of Sox9 depleted embryos, which suggests the function of Sox9 in neural crest specification [17]. Overexpression studies on Sox9 cause ectopic expression of several of early neural crest markers, consistent with an early neural crest formation [18]. Depletion of Sox9 protein in Xenopus whole embryos using morpholino antisense oligonucleotides causes a dramatic loss of the early otic placode markers [15].
Despite the data available regarding the expression and functional analyses of Sox9 in the induction of neural crest and otic placode, there have been no dose dependent regulations of Sox9 during Xenopus embryogenesis. Here it is reported the regulation of Sox9 in the development of neural crest and otic placode in a time and dose dependent fashion.
Materials and Methods
Xenopus laevis were handled in accordance with animal welfare regulations of Institutional Animal Care and Use Committees (IACUC), Chonbuk National University Laboratory Animal Centre, South Korea. Embryos were obtained from adult Xenopus laevis by standard hormone-induced egg laying and artificial fertilization. All efforts were made to minimize the discomfort of animal used.
Hormone-inducible constructs were generated by sub-cloning the coding region of Sox9 gene into pCS2 + GR [19]. Fusion constructs subcloned into pCS2+, referred as Sox9-GR, were sequenced, and the corresponding protein was monitored using an in vitro transcription/translation coupled rabbit reticulocyte lysate system (Promega, Madison, WI). Sox9-GR mRNA was synthesized in vitro by using Message Machine kit (Ambion, Austin, TX).
Synthetic Sox9-GR mRNA was injected into the animal pole of one blastomere at the two-cell stage. Embryos were also co-injected with β-galactosidase mRNA (β-gal, 1 ng). Sox9-GR injected embryos were cultured in 0.1 × normal amphibian medium (NAM) containing 10 μM dexamethasone from stage 14 or stage 17. Siblings injected with these constructs and cultured in the absence of dexamethasone were used as control.
Embryos at the appropriate stage were fixed in MEMFA and successively processed for Red Gal staining (Research Organics) to identify the injected side and in situ hybridization. Antisense DIG-labeled probes (Genius kit, Roche) were synthesized using template cDNA encoding Pax8 [20] and Snail2 [21] for in situ hybridization. Whole-mount in situ hybridization was performed as previously described [22]. For in situ hybridization on sections, embryos were fixed in MEMFA for 1 hr and embedded in Paraplast. Sections (12 μm) were then cut on a rotary microtome and hybridized with the appropriate probes as described previously [23]. Sections were then briefly counterstained with eosin.
Results
Using whole mount in situ hybridization the expression patterns of Sox9 were analyzed in developing Xenopus. At neurula stage, Sox9 was expressed both in the neural crest and otic placode domain (Fig. 1A). Tissue sections of stage 15 embryos highlighted Sox9 expression in the neural crest as well as in the prospective otic placode that appears to be confined to the sensory layer of the ectoderm (Fig. 1B). By the tailbud stage, the diffuse expression of Sox9 in the presumptive otic placode was more confined to the otic vesicle and branch arches (Fig. 1C). To determine the tissue specific expression analysis of Sox9 gene, whole mount in situ hybridization for both Snail2 and pax8 was performed in staged matched embryos. Both Sox9 and Snail2 were expressed in the develraloping neura crest (Figs. 1A and 1D), and Sox9 shared a common expression domain with Pax8 in the prospective otic placode (Figs. 1A, 1E and 1F).
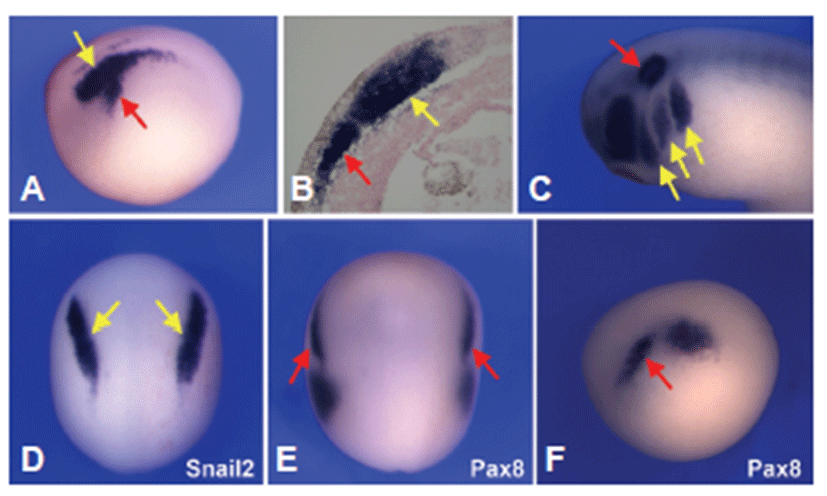
To evaluate the role of Sox9 in the induction of neural crest and otic placode, a hormone-inducible version of Sox9 was injected in the two-cell stage embryos in which Sox9 was fused to the hormone-binding domain of human glucocorticoid receptor (Sox9-GR). The activity of this fusion protein can be regulated by addition of dexamethasone to the embryo culture medium [24]. Embryos injected with 0.05 ng, 0.2 ng or 0.5 ng of Sox9-GR mRNA were treated with dexamethasone at late gastrula stage (stage 10.5) or early neurula stage (stage 14) and analyzed for gene expression at late neurula (stage 17) or early tailbud (stage 22) stage. Overexpression of Sox9 (0.05 ng) at the gastrula stage before induction of neural crest and otic placode resulted in an expansion of the both neural crest specific marker gene Snail2 and otic placode marker gene Pax8 expression at late neurula stage and at early tailbud stage, respectively (Figs. 2B and 2C). Sox9 overexpression (0.2 ng) at gastrula stage was consistent with the expression at low (0.05 ng) dose but at higher dose (0.5 ng) expression of both Snail2 and Pax8 was reduced or lost (Figs. B2 and 2C).
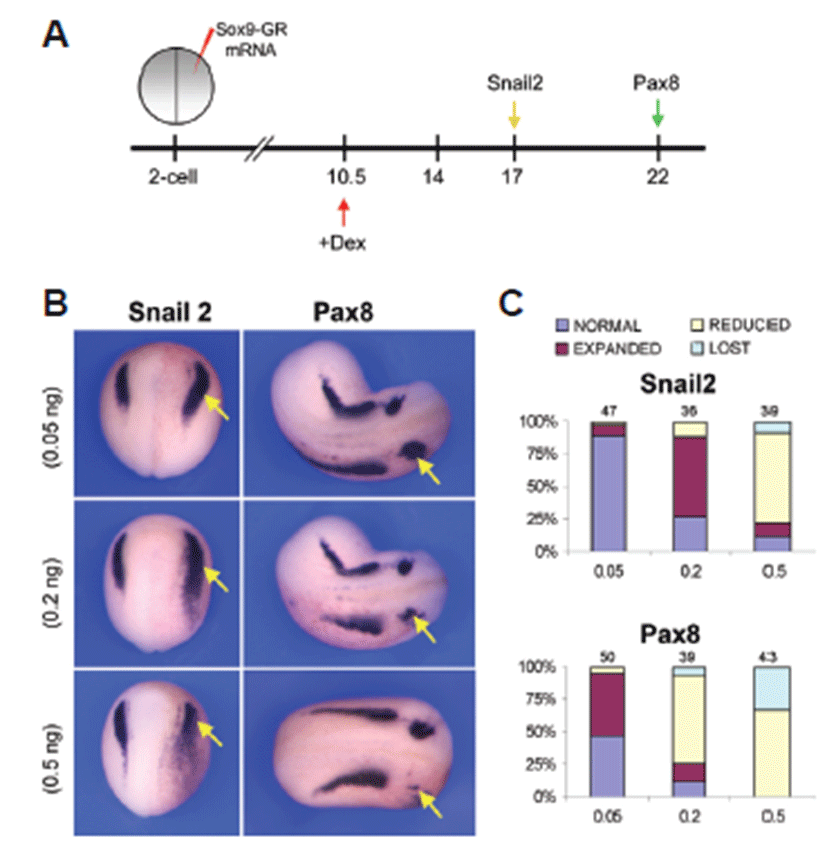
Over expression of Sox9 at gastrula stage before induction of neural crest and otic placode showed an interesting change. At stage 17, unilateral injection of low dose of Sox9-GR mRNA (0.05 ng) resulted in almost normal expression (89.4%, n=47) of Snail2 in neural crest, whereas injection of the mid-dose level of (0.2 ng) Sox9-GR resulted in a marked expansion of Snail2 expression in more than 60% (n=36) of the embryos analyzed. But higher dose (0.5 ng) of Sox9 overexpression showed a different scenario that the expression of Snail2 was mostly reduced (69.2%, n=39) (Fig. 2C). In case of injection of Sox9-GR mRNA at lower dose (0.05 ng), the otic placode marker Pax8 was expanded in 48% (n=50) of the embryos but at the mid-dose level (0.2 ng) of Sox9-GR, Pax8 expression was reduced in 69.2% (n=39) of the embryos at stage 22. At higher dose (0.5 ng), Pax8 expression was mostly reduced or lost in Sox9-overexpressed embryos (67.4%, n=43 and 32.6%, n=43, respectively) (Fig. 2C).
After induction of neural crest cells and otic placode the expression of Snail2 and Pax8 showed a different level of expression in the embryos analyzed. In this case, injection of 0.05 ng Sox9-GR mRNA resulted in almost normal expression of Snail2 in 94% (n=50) of the embryos whereas at 0.2 ng dose level Snail2 expression was mostly expanded in 74.1% (n=27) of the embryos and at the higher dose level consistent with the expression of 0.2 ng dose level (78.8%, n=33) (Figs. 3B and 3C). Pax8 showed almost normal expression in 79.1% (n=43) of the embryos injected with lower dose (0.05 ng) of Sox9-GR but injection of 0.2 ng Sox9-GR resulted in expansion of Pax8 expression in 37% (n=54) of the embryos. 55% of higher dose Sox9-GR injected embryos (n=40) exhibited a reduction of Pax8 expression in the otic placode (Figs. 3B and 3C).
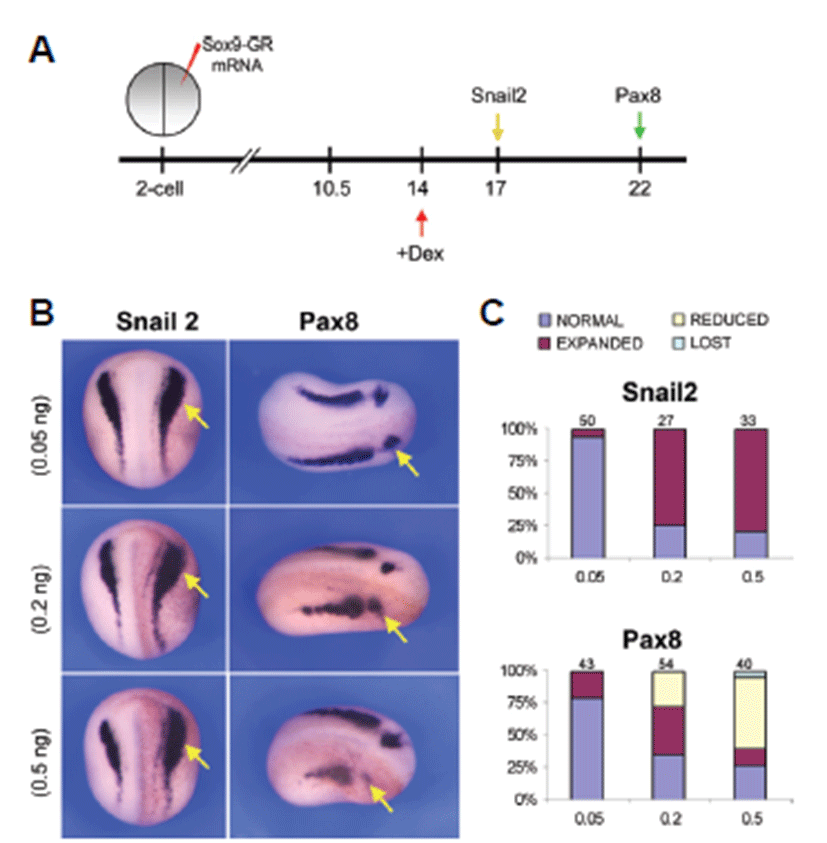
Discussion
Head skeleton and sensory ganglia originate from two embryonic structures: the neural crest and the cranial placodes. Neural crest arises from the entire length of the neural tube starting form a region posterior to the prospective diencephalon and will develop a broad cell types including pigment cells, cartilage and smooth muscle cells. Craniofacial sensory organs arise from placodal structures located at the periphery of the neural plate [1, 25]. The inner ear is derived from a simple patch of thickened ectoderm, the otic placode that arises next to the posterior hindbrain [26-29]. Previous studies suggest the role of hindbrain in otic placode induction and, members of the fibroblast growth factors (Fgf) family play a crucial role in this induction in several species [30-33]. Fgf originated paraxial mesoderm is also required for the induction of neural crest [34]. Wnt signaling has also been proposed to participate in otic placode induction and early neural crest induction. Both signals control and regulate the signaling in a dose dependent manner. In Xenopus Sox9 is a downstream of Wnt and Fgf, and both of them control Sox9 regulations regarding the development of neural crest and otic placode [33-35].
In this study it was found that at early neurula stage, Sox9 was highly expressed in the migrating neural crest and otic placode domain which were located at the periphery of the neural plate. By the tailbud stage, the expression was confined to the otic vesicle and branchial arches which arose from the neural crest cells. This result is consistent with Sox9 requirements for the maintenance of progenitors in the otic vesicle [36]. Sox proteins are known to regulate their target genes through interaction with cell specific partner factors [37]. The expansion of the neural crest and otic placode in Sox9-GR injected embryos could reflect the inability of the embryo to regulate the proper level of neural crest marker gene Snail2 and the otic placode specific gene Pax8.
Though it is not clear how Sox9 controls the positional genes that lead to neural crest and otic placode formation, Sox9 controls the development of dorsal and ventral part of otic vesicle in a dose dependent manner [36]. Sox9 differently interferes the expression of neural crest and otic placode specific genes. At the same dose level of Sox9, the expression of otic placode specific gene more severely affected than neural crest marker gene. In the present study, in low dose of Sox9-GR mRNA injected embryos, Snail2 expression was mostly normal and reduced or expanded with the increasing dose level. But too higher dose may reduce or lose the expression of neural crest marker genes.
Sox9 overexpression affected the expression of neural crest marker and otic placode marker in both time and dose dependently. The expression of neural crest marker gene, Snail2 and otic placode marker gene, Pax8 showed a different phenotype when Sox9 was overexpressed either before induction of neural crest and otic placode (stage 10.5) and after induction but before specification (stage 14). After cellular induction lower dose of Sox9-GR mRNA injection resulted in almost normal expression of Snail2 whereas Pax8 was expanded in more than 50% of the embryos. It may be related with the timing of induction and differentiation of the otic placode. Placodes derive from a common pre-placodal domain consisting of a narrow band of ectoderm that encompasses the anterior neural plate that is established at the end of gastrulation [1, 25, 38, 39]. Cranial placodes form in two steps. The first step is the acquisition of a general placodal state (pan-placodal domain) in the ectodermal domain adjacent to the neural ectoderm, with the second step being the determination of the identity of each placode by local inducing signals [40]. Due to a timing of induction of neural crest, Snail2 was not affected acutely whereas Pax8 was expanded due to the differentiation and specification of otic placode. And in higher dose, neural crest was largely expanded, but Pax8 was mostly reduced, which suggested that Sox9 controlled the genes expressed in the neural crest and otic placode domain in a time and dose dependent manner.
Therefore, it should be proposed that Sox9 is an important regulator for the maintenance of neural crest and otic placode formation and mediation of cell fate where it controls the signaling pathway in a time and dose dependent fashion.