Introduction
With rapid globalization of food production and trade, many outbreaks of foodborne diseases now take place on worldwide dimensions. Foodborne disease resulting from consumption of food contaminated with pathogenic bacteria and/or their toxins is a vital concern to public health [1].
In general, it is estimated that up to one-third of the population for industrialized countries suffer a foodborne illness each year [2]. In the United States, Centers for Disease Control and Prevention (CDC) estimates that each year roughly 1 in 6 Americans (or 48 million people) get sick, 128,000 are hospitalized, and 3,000 die of foodborne diseases. Although the vast majority of cases are mild, a significant number of deaths do occur and the high levels of acute infections and chronic sequela lead to billions of dollars in medical costs and lost productivity [3]. According to the data of Ministry of Food and Drug Safety during 2006~2012 [4], the number of foodborne disease occurrences and patients was 2,137 cases and 54,386 persons, respectively. Pathogenic Escherichia coli (E. coli), Norovirus, Staphylococcus aureus (S. aureus), nontyphoidal Salmonella and Vibrio parahemolyticus were the top five pathogens causing domestically acquired foodborne illnesses in Korea.
S. aureus is one of the major bacteria causing foodborne disease. The bacterium is a gram positive coccus bacterium that is a member of the Firmicutes, and is frequently found in the human respiratory tract and on the skin. Foodborne disease-associated strains often promote infections by producing potent protein toxins and expressing cell-surface proteins that bind and inactivate antibodies [5]. In addition, E. coli O157:H7 is an enterohemorrhagic serotype of the bacterium E. coli and a cause of foodborne illness, typically through consumption of contaminated food [6].
With the investigation of the bacterial safety for meats, fishery and vegetables in the local market by Korean Consumer Agency [7], S. aureus and E. coli were detected at 27.8 and 62.7% of 212 cases surveyed, respectively, and among them, 94.8% of S. aureus and 92.9% of E. coli were identified as antibiotic-resistant bacteria.
Around the world, the widespread use of antibiotics in food animal production systems has resulted in the emergence of antibiotic-resistant bacteria that can be transmitted to humans through the food chain [8]. Infection with antibiotic-resistant bacteria negatively impacts on public health, due to an increased incidence of treatment failure and severity of disease [9].
In previous studies, the antimicrobial properties of several plant-derived essential oils, and a variety of active components of these oils have been identified [10-14]. Carvacrol (CV) and thymol, the two main phenols that constitute about 78~85% of oregano oil, are mainly responsible for the antibacterial activity of the oil obtained from Origanum vulgare L. [15].
Generally recognized as a safe food additive, CV is used as a flavoring agent in several products [16, 17]. Moreover, this natural phytochemical has a broad-spectrum antimicrobial activity and has not induced resistance in gram-positive and gram-negative bacteria after prolonged exposure [18, 19].
Milk is a complex medium in which lipophilic proteins reduce the bioavailability due to the potential interaction with antimicrobial compounds [20]. Therefore, milk was chosen as an in vitro model for studying the antimicrobial potential of CV for controlling foodborne pathogens.
The present study was to determine the efficacy of CV for killing S. aureus and E. coli O157:H7 in milk. Specifically, the antimicrobial effect of CV was investigated on each of S. aureus and E. coli O157:H7 as serious bacteria causing foodborne disease.
Materials and Methods
CV (99%) and gentamicin sulfate were purchased from Sigma-Aldrich Korea Ltd. (Yongin, Korea). A solution was prepared by dissolving 1.0 g of CV in enough ethanol (≥99.8%) to make 10 mL of stock solution and by dissolving 1.0 mg gentamicin sulfate in sufficient distilled water to make 10 mL of stock solution. Stock solutions were diluted with absolute ethanol prior to use. Fresh raw milk free from antibiotic residues was collected from the bulk tank at Gyeongsang National University dairy farm and autoclaved at 121°C for 15 min.
S. aureus (KVCC-BA11000330) and E. coli O157:H7 (KVC-BA0701446) originated from dairy cattle were obtained from the Korea Veterinary Culture Collection (KVCC, Anyang, Korea). All bacteriological media used in the study were purchased from Becton Dickinson Korea Ltd. (Seoul, Korea). The purity of each culture was ensured by characteristic morphology on mannitol salt agar (S. aureus) and sorbitol MacConkey agar (E. coli O157:H7). For the preparation of inocula, each bacterium was grown separately in 10 mL of tryptic soy broth (TSB) for 24 hr at 37°C. The cells were then sedimented by centrifugation (3,600 × g for 15 min at 4°C), washed twice with sterile phosphate buffered saline (PBS) (pH 7.2), and resuspended in PBS. The populations of each bacterium were determined by plating 0.1-mL portions of appropriate dilutions on tryptic soy agar (TSA) plates and incubating the plates at 37°C for 24 hr. After incubation, the bacterial concentration was represented as colony forming unit (CFU)/mL.
The MIC and MBC of CV against each bacterial pathogen were determined by a broth dilution assay described by the previous study [21]. Milk tubes containing CV in the range of 0 to 50 mg/mL (2-fold dilution) were inocu1ated separately with each bacterial pathogen at 6.0 log CFU/mL and incubated at 37°C for 24 hr. Control samples included milk inocu1ated with each pathogen. Following incubation, the samples were serially diluted (1:10) in PBS and appropriate dilutions were spread on TSA plates. The plates were incubated at 37°C for 24 hr. The lowest concentration of antimicrobial treatment that inhibited visible growth of the pathogen after incubation was taken as MIC of the treatment. The lowest concentration of the treatment that prevented growth of the organism after subculture on TSA following serial dilution and plating was taken as MBC. Triplicate samples were included for each treatment, and the experiment was replicated three times.
The bactericidal kinetics of CV were studied by inoculating sterile milk containing MIC, MBC, and 2 × MBC of CV with each S. aureus and E. coli O157:H7 at 6.0 log CFU/mL. Negative and positive controls were inoculated aseptic milk with no added antimicrobial and supplemented with gentamicin sulfate at a dose of MBC, respectively. The samples were incubated at 37°C for 24 hr. Surviving populations of each bacterial pathogen were enumerated at 0, 6, 12, and 24 hr of incubation by plating 0.1-mL portions of the samples directly or after serial dilutions (1:10 in PBS) on duplicate TSA plates. Pathogens that were not detected by direct plating were tested for surviving bacteria by enriching 1 mL of the sample in 100 mL of TSB at 37°C for 24 hr. When growth was observed in the broth, the culture was streaked on TSA plates and observed for typical colonies of each pathogen. Each treatment was done in duplicate and the experiment was replicated three times.
Milk samples containing each of MBC or 2 × MBC of CV were inoculated with each of S. aureus and E. coli O157:H7 at 6.0 log CFU/mL and incubated at 37°C for 14 days. Inoculated milk samples containing no CV served as controls. The surviving bacteria were determined immediately after CV addition and at 24-hr intervals until day 14. To mimic the bacterial survival after CV addition, approximate 6.0 log CFU/mL of each S. aureus and E. coli O157:H7 were inoculated into the same milk samples every 48 hr until day 6 (day 2, 4, and 6) and bacterial populations were determined on TSA after 24 hr. Duplicate samples were inoculated and the experiment was replicated three times.
The data were expressed as the means ± standard deviation (S.D.) for the triplicate experiments. The significance between the control group and experimental groups was determined by Student’s t-test. A difference at the level of P<0.05 was considered to be statistically significant.
Results
MIC and MBC of CV against S. aureus and E. coli O157:H7 are provided in Table 1. Against S. aureus, MIC and MBC of CV were 15.0 and 20.0 mg/mL, respectively, and those of CV against E. coli O157:H7 were 16.0 and 32.0 mg/mL, respectively. However, MIC and MBC of gentamicin were both 0.25 μg/mL against S. aureus and E. coli O157:H7. The average pH of milk with no addition of CV was slightly higher than that of milk with addition of CV.
Compounds |
MIC |
MBC |
||
---|---|---|---|---|
S. aureus | E. coli O157:H7 | S. aureus | E. coli O157:H7 | |
|
||||
Carvacrol (mg/mL) | 15 | 16 | 20 | 32 |
Gentamicin (µg/mL) | 0.25 | 0.25 | 0.25 | 0.25 |
The bactericidal kinetics of CV on the foodborne pathogens in milk are depicted in Fig. 1. The average initial bacterial population in all the treatment and control samples for the two foodborne pathogens was approximate 6.0 log CFU/mL. In the control samples, the bacterial population increased during a 24-hr incubation period. In the treatment samples containing CV at MIC (15.0 mg/mL), MBC (20.0 mg/mL) and 2 × MBC (40.0 mg/mL), the number of S. aureus was significantly reduced at 24 (P<0.05), 12 (P<0.05) and 6 hr (P<0.001) post-incubation, respectively, compared with that of control. The presence of CV at MBC and 2 × MBC reduced the population of S. aureus to undetectable levels in 24 and 12 hr, respectively. In addition, the population of E. coli O157:H7 treated with MIC (16.0 mg/mL), MBC (32.0 mg/mL) and 2 × MBC (64.0 mg/mL) of CV was significantly reduced at 24 (P<0.05), 6 (P<0.05) and 6 hr (P<0.001) post-incubation, respectively, compared with that of control. With the CV at MBC and 2 × MBC, the population of E. coli O157:H7 reduced to undetectable levels in 24 and 12 hr, respectively. With the results of this study, the concentration of CV at MBC completely inactivated each bacterial pathogen by 24 hr of incubation. As expected, for all bacteria, CV at MIC concentration did not allow growth to occur.
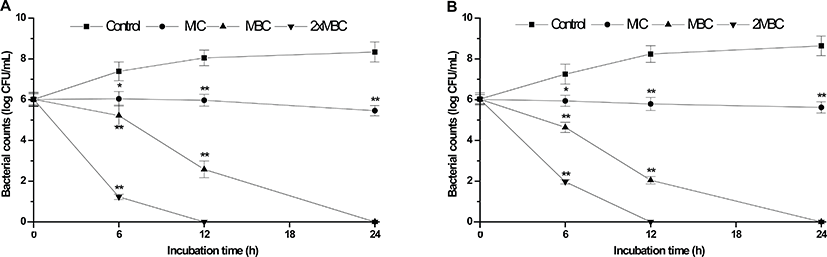
The results from an experiment determining the persistence of antimicrobial activity of CV in milk are depicted in Fig. 2. S. aureus and E. coli O157:H7 (6.0 log CFU/mL) inoculated in the milk samples on day 0 were completely inactivated by each 2 × MBC of CV after 24 hr. On day 3 and 6.0 log CFU/mL of S. aureus and E. coli O157:H7 reinoculated into the milk samples on day 2 were reduced to less than 1.0 log CFU/mL. On day 4, S. aureus and E. coli O157:H7 populations reinoculated at 6.0 log CFU/mL into the milk samples was reduced to less than 2.0 log CFU/mL after 24 hr. This trend in bacterial reduction was observed on the subsequent days, where 6.0 log CFU/mL of S. aureus and E. coli O157:H7 inoculated on day 6 were decreased to undetectable levels on day 12 of the experiment. A similar trend in S. aureus and E. coli O157:H7 inactivation was also observed in milk samples containing each MBC of CV, but the magnitude of killing was slightly less than that observed with each 2 × MBC of CV. In control samples, all the population of S. aureus and E. coli O157:H7 increased to over than 7.0 log CFU/mL by 24 hr.
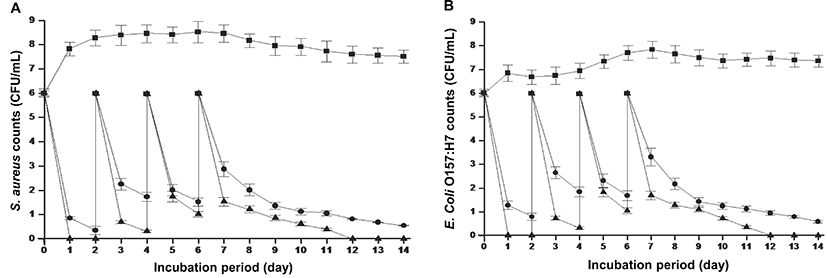
Discussion
Antibiotic administration is still widespread in many underdeveloped and developing countries and some antibiotics are accepted as global food sources. Additionally, it became more significant that the globalization of food consumption habits raises some foodborne pathogens in developed countries of which infection has not been reported before [23].
Since 1999, European Union banned antibiotic administration for promoting the growth of farm animals. In many developed countries, antibiotic application is a question of concern. There is an awareness about the antibiotic-resistant bacteria and their effects on public health [24].
As the widespread use of antibiotics in food animal production systems has resulted in the emergence of antibiotic-resistant zoonotic bacteria that can be transmitted to humans through the food chain, the development of alternative strategies using compounds not subject to limitations associated with antibiotics is needed [9, 22]. In the present study, the data are presented for the efficacy of CV for killing foodborne pathogens in vitro.
In the previous studies, antibacterial activity of CV has been demonstrated against both gram-positive and gram-negative pathogens, including Salmonella thyphimurium, E. coli O157:H7, Camphylobacter jejuni, and Listeria monocytogenes [25-27]. Several studies have reported a decreased antimicrobial effect of plant extracts when used in foods or complex systems [28-30]. A higher concentration of plant-derived components is required to achieve the same antimicrobial activity in complex foods such as meat, fish, dairy products, and vegetables than in microbiological media [10, 31]. Similarly, the milk composition, especially fat level, reduces the antibacterial efficacy of components derived from plants [29]. In light of the above findings, MIC and MBC of CV in this study were determined against foodborne pathogens directly in milk rather than in any synthetic laboratory media.
The antibacterial mechanism of the action of CV with a hydrophobic property is the disruption of the lipid-containing bacterial cytoplasmic membranes, which increase its permeability and depolarizes its potential [32, 33]. Besides the interaction with cytoplasmic membranes, CV has been proposed to interact with membrane proteins and periplasmic enzymes [34].
To be an effective antimicrobial agent as a therapy of foodborne disease under clinical conditions, CV should maintain its antimicrobial activity over an extended period. However, MIC and MBC experiments and time-kill assay determined the antimicrobial activity of CV in milk only up to 24 hr. Moreover, CV cou1d be degraded or inactivated in milk over a period of time. Therefore, the persistence of antimicrobial activity of CV on S. aureus and E. coli O157:H7 was determined in milk for 14 days to confirm if CV could maintain antimicrobial activity over this period. S. aureus and E. coli O157:H7 were chosen for this experiment because these bacteria are a common cause of food poisoning [35].
Therefore, to investigate whether CV would maintain its effectiveness over a period of time in a milk environment, S. aureus and E. coli O157:H7 were reinoculated into milk samples on day 2, 4, and 6 of the experiment after the addition of CV on day 0. As observed in Fig. 2, the antimicrobial effect of CV persisted for the duration of the experiment without any loss of activity. It was also found that CV added to milk on day 0 was effective in killing large populations of S. aureus and E. coli O157:H7 inoculated multiple times on subsequent days.
Results from the present study suggest that CV may be useful as an alternative to antibiotics for the control of foodborne pathogens. Although CV may be applicable in controlling foodborne pathogens in milk, future experiments are needed to determine the antibacterial activity of CV in various foods.